Table of Contents
- Introduction
- Introduction
- Application Information
- Presets
- Control Parameters
- Inputs
- System
- Tables
- Features
- Technical Specifications
- FAQ
- Setup ``Tips and Tricks''
- Troubleshooting
- Some features in the BeoLab 50 interface are disabled
- Lip Sync problems
- Echo problems
- Loudspeakers don't turn on automatically
- Loudspeakers never shut off
- The BeoLab 50 interface doesn't work
- Loudspeakers are distorting
- Loudspeakers are noisy / too quiet
- USB Audio not working
- S/P-DIF input not working
- Optical input not working
- Automatic switching of inputs not behaving as expected
- Appendix 1: Recommendations for Critical Listening
- Appendix 2: Introduction to Parametric Equalisers
- Appendix 3: The Influence of Listening Room Acoustics on Loudspeakers
- Appendix 4: Loudspeaker Directivity and Distance Perception in Stereo Imaging
- Appendix 5: Microphone placement strategy when creating ARC Zones
- Appendix 6: ABL - Adaptive Bass Linearisation
- Appendix 7: Thermal Compression Compensation
- Appendix 8: Control of BeoLab 50 using the BeoRemote 1
- Index
Bang & Olufsen BeoLab 50 User Manual
Displayed below is the user manual for BeoLab 50 by Bang & Olufsen which is a product in the Loudspeakers category. This manual has pages.
Related Manuals
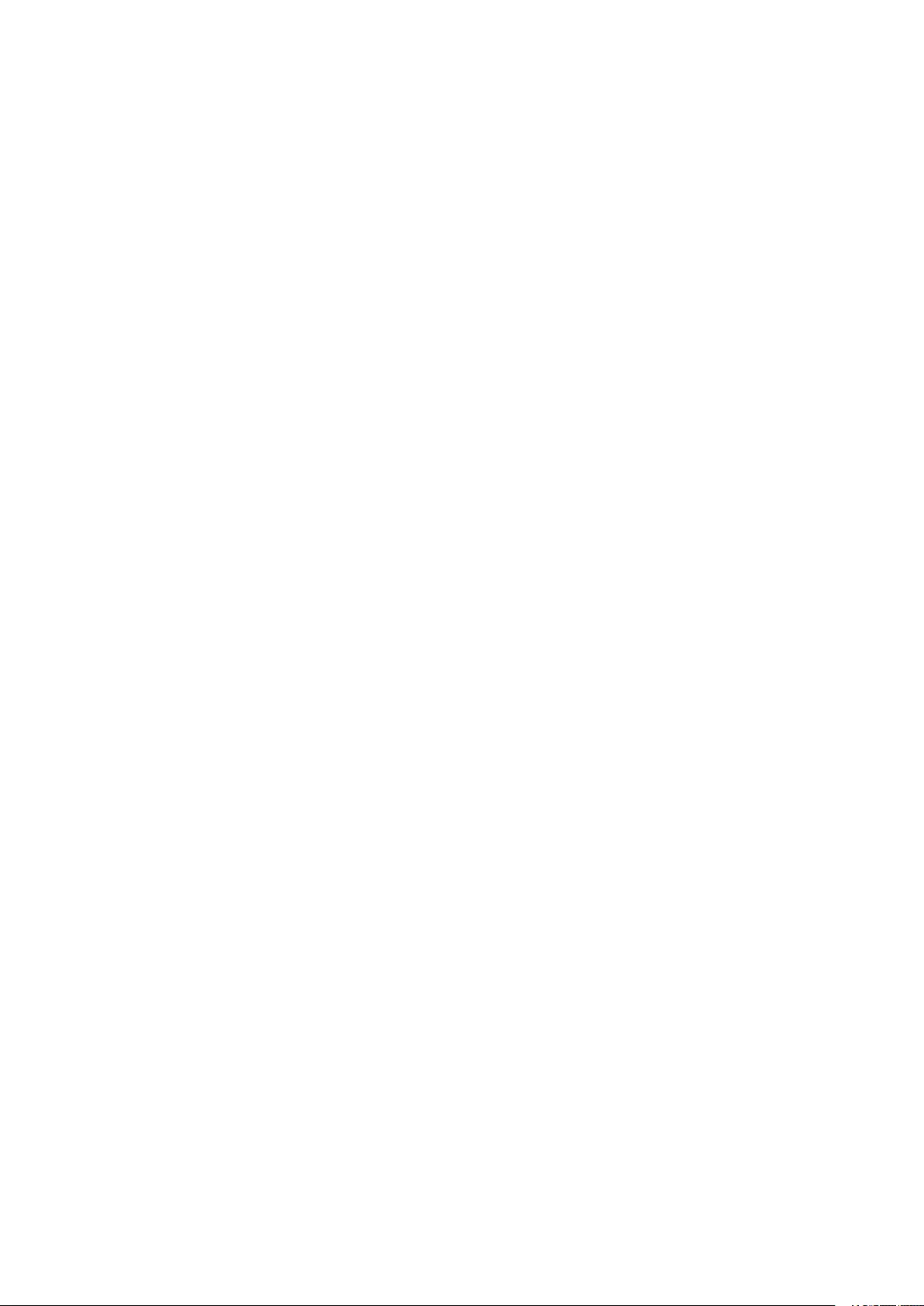
BeoLab 50
Technical Sound Guide
Bang & Olufsen A/S
September 21, 2017
Please note that text in grey indicates features that are not yet released
(SW Version 2.1.0.6242)
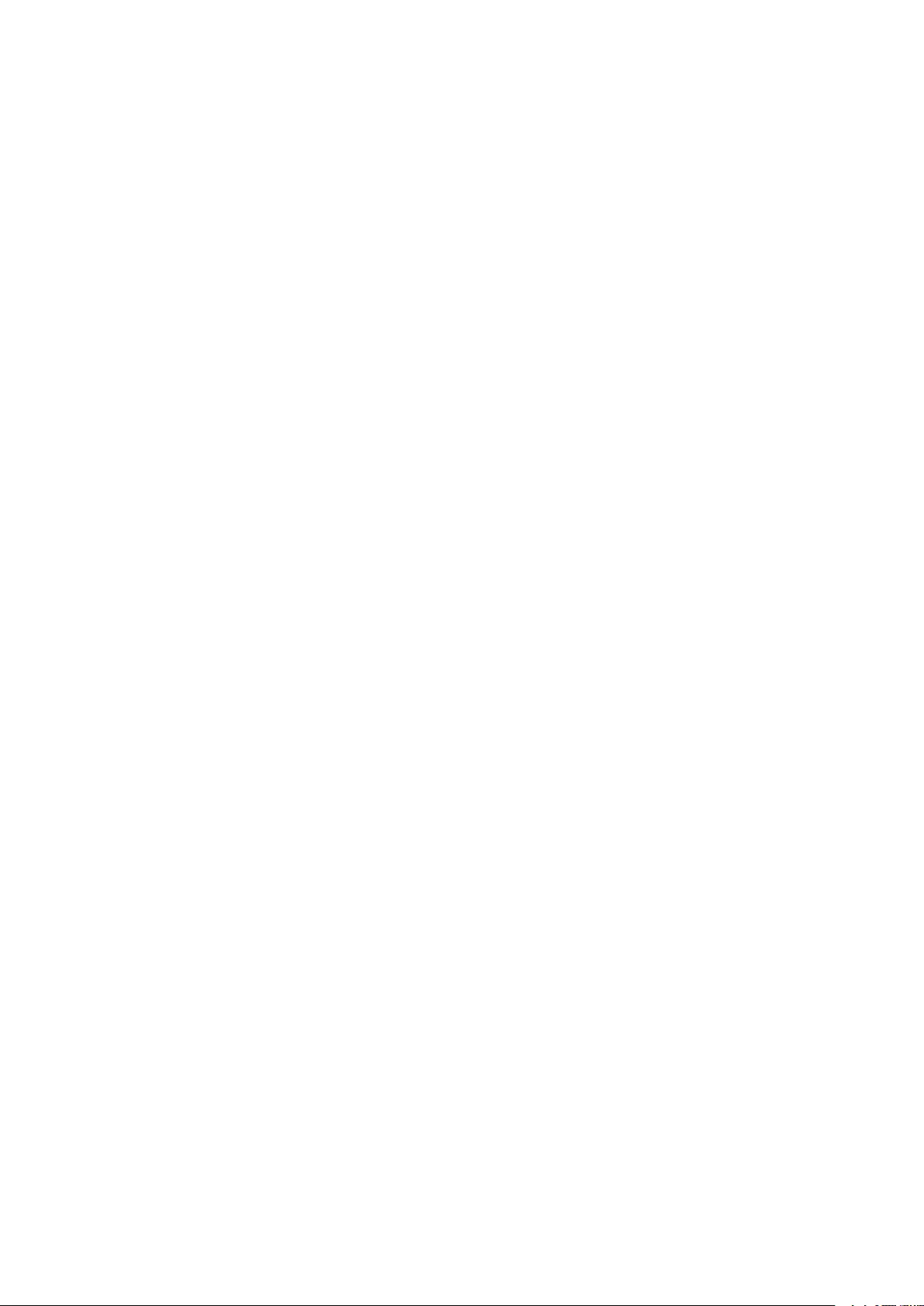
Contents
1 Introduction 7
1.1 Features ............................................................. 7
1.1.1 Beam Width Control .................................................... 7
1.1.2 ARC: Active Room Compensation ............................................. 8
2 Application Information 9
2.1 Menu Navigation ........................................................ 9
2.2 Menu Map ............................................................ 10
3 Presets 11
3.1 What is a Preset? ........................................................ 11
3.2 Preset management ...................................................... 11
3.2.1 Selecting a Preset ..................................................... 11
3.2.2 Creating a Preset ..................................................... 11
3.2.3 Editing a Preset ...................................................... 11
3.2.4 Deleting a Preset ..................................................... 11
4 Control Parameters 12
4.1 Beam Width Control ...................................................... 12
4.1.1 Narrow ........................................................... 12
4.1.2 Wide ............................................................ 13
4.1.3 Comment .......................................................... 14
4.2 Speaker Distance ........................................................ 14
4.2.1 Adjusting Speaker Distances for more than one listening position ........................... 14
4.2.1.1 Automated Measurement of Speaker Distance ................................ 14
4.3 Speaker Level .......................................................... 14
4.4 Speaker Role .......................................................... 14
4.5 Active Room Compensation .................................................. 15
4.5.1 Creating a new ARC Zone ................................................. 15
4.5.2 Selecting an ARC Zone .................................................. 15
4.5.3 Combining ARC Zones ................................................... 15
4.6 Volume ............................................................. 16
4.7 Mute .............................................................. 16
4.8 Tone Controls .......................................................... 16
4.8.1 Treble ............................................................ 16
4.8.2 Bass ............................................................ 16
4.9 Advanced Controls ....................................................... 17
4.9.1 Latency Mode ....................................................... 17
4.9.1.1 Auto ....................................................... 17
4.9.1.2 High ....................................................... 17
4.9.1.3 Low ........................................................ 17
4.9.1.4 Effects of Latency Mode on Beam Width .................................... 18
4.9.2 Loudness .......................................................... 18
4.9.3 Frequency Tilt ....................................................... 18
2
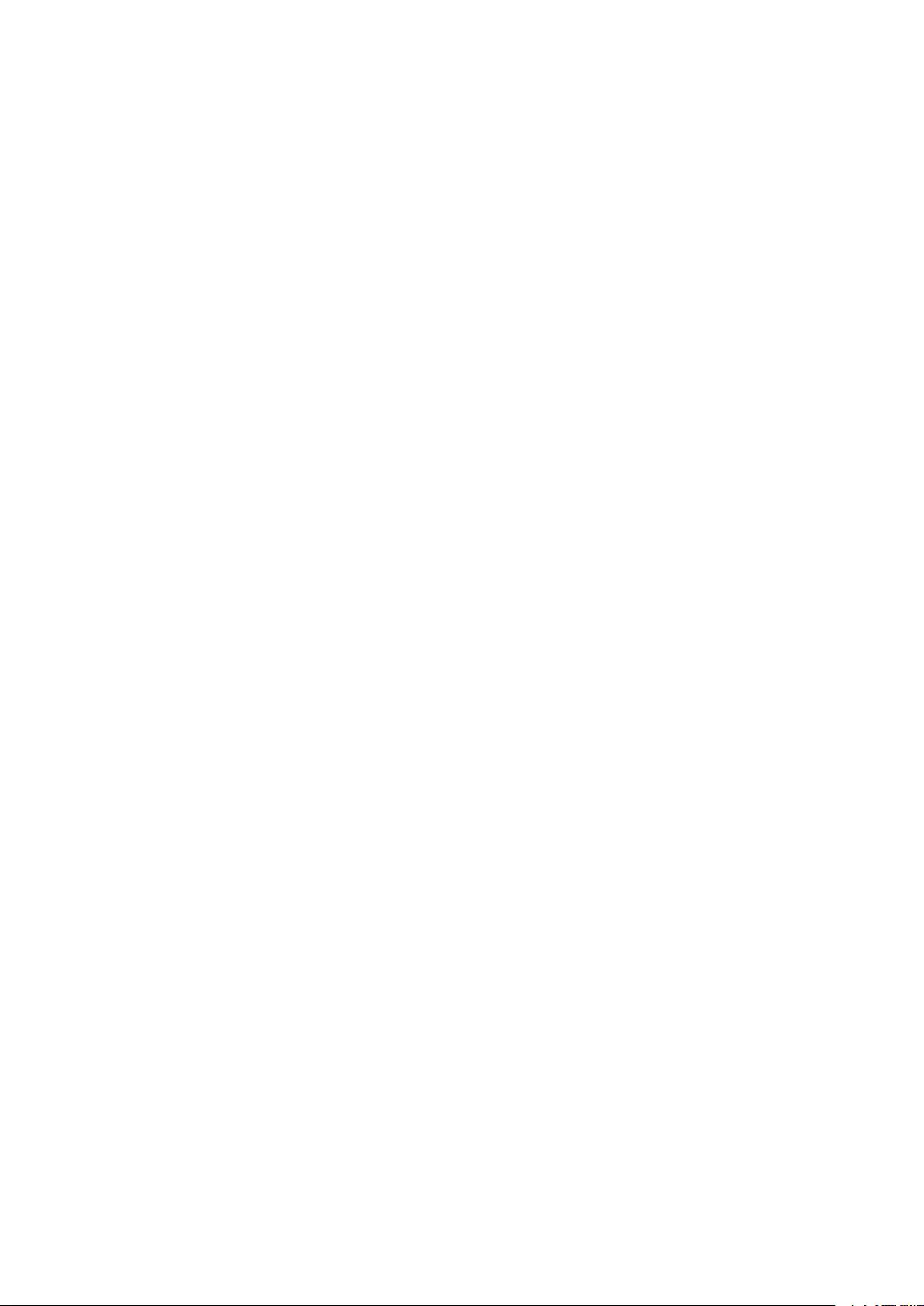
4.9.4 Sound Enhance ...................................................... 18
4.9.5 Sound Design ....................................................... 19
4.9.6 Parametric Equaliser .................................................... 19
4.9.6.1 Magnitude Response Plots ........................................... 19
4.10 Automating Preset Selection .................................................. 20
4.10.0.1 By Speaker Group ................................................ 20
4.10.0.2 By Source .................................................... 21
4.10.0.3 Mixed systems .................................................. 21
5 Inputs 23
5.1 Inputs Selection ........................................................ 23
5.1.1 Automatic Selection .................................................... 23
5.1.1.1 Selection Priority ................................................. 23
5.1.2 Manual Selection ...................................................... 23
5.2 Individual Input Parameters .................................................. 23
5.2.1 Re-naming ......................................................... 23
5.2.2 Gain Offset ......................................................... 24
5.2.3 Detection Threshold .................................................... 24
5.2.4 Auto-detection ....................................................... 24
5.2.5 Maximum Input Voltage .................................................. 24
5.2.6 Time-out .......................................................... 24
5.2.7 Input Impedance ...................................................... 25
5.2.8 Control Volume of S/P-DIF (or Optical) input using Power Link ............................. 25
5.2.9 USB Volume enabled ................................................... 25
5.3 Connection Panels ....................................................... 25
6 System 27
6.0.1 About ............................................................ 27
6.0.2 Max Volume ........................................................ 27
6.0.3 Startup Volume ...................................................... 27
7 Tables 28
7.1 Loudspeaker Sensitivity .................................................... 28
7.2 Volume Control ......................................................... 28
7.3 Parametric Equaliser ...................................................... 28
8 Features 29
8.1 Resonance-based Sound Design ................................................ 29
8.2 Phase-Optimised Filtering ................................................... 29
8.3 Automatic Bass Linearisation (ABL) and Thermal Protection ................................. 29
8.4 Thermal Compression Compensation ............................................. 30
8.5 Production “Cloning” ...................................................... 30
9 Technical Specifications 31
9.1 Total System .......................................................... 31
9.2 Preamplifier and Processor Section .............................................. 31
3
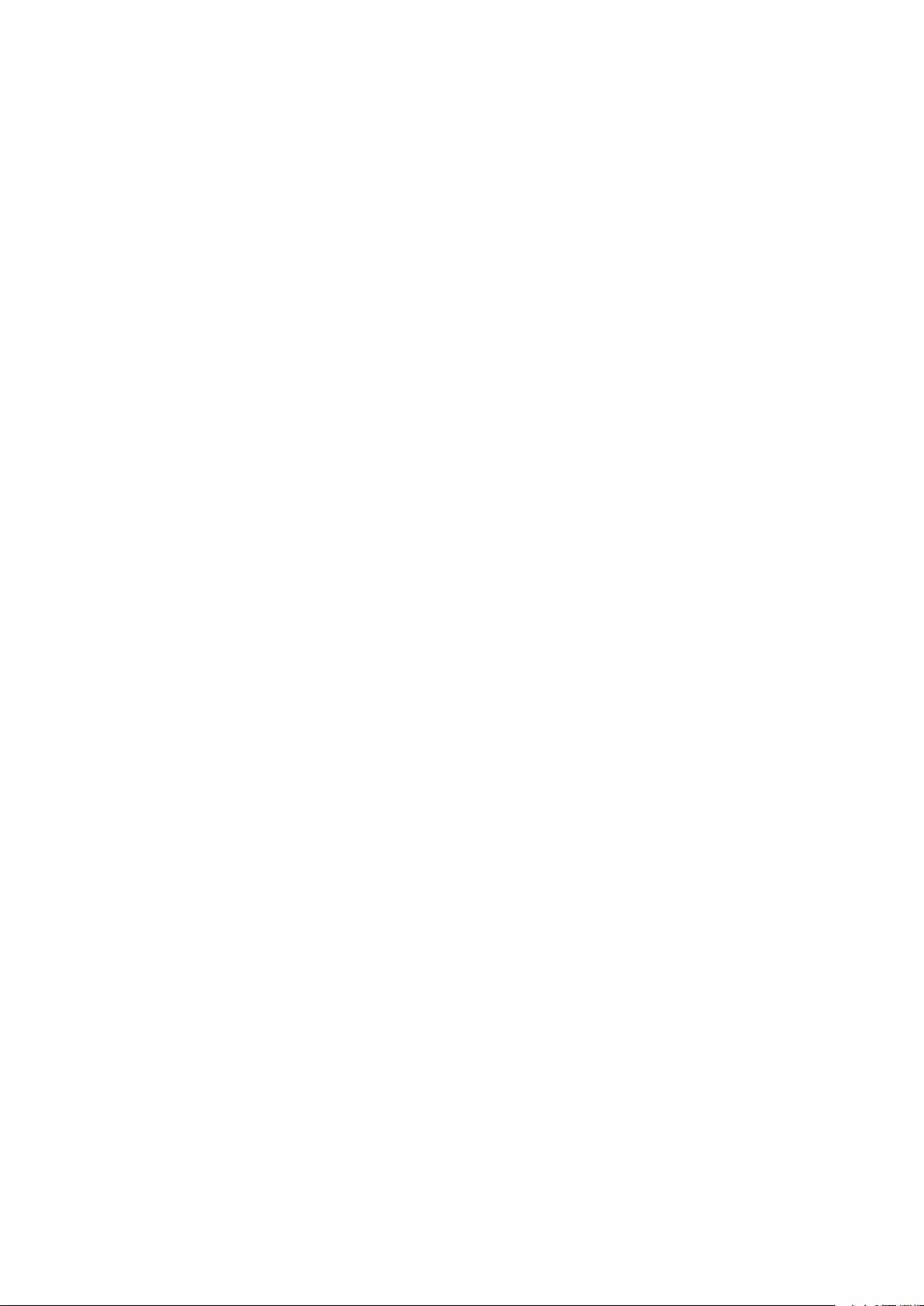
9.2.1 Overall Specifications ................................................... 31
9.2.1.1 Digital input to DAC outputs .......................................... 31
9.2.1.2 Analogue input to DAC outputs ......................................... 31
9.2.2 Inputs ............................................................ 32
9.2.2.1 Analogue Inputs ................................................. 32
9.2.2.2 Digital Inputs ................................................... 32
9.2.3 Digital Signal Processor .................................................. 33
9.2.4 Digital to Analogue Converters .............................................. 33
9.3 Power Amplifiers ........................................................ 34
9.4 Loudspeaker Drivers ...................................................... 34
9.4.1 Tweeter ........................................................... 34
9.4.2 Midranges ......................................................... 34
9.4.3 Woofers .......................................................... 34
9.5 Digital Power Link ....................................................... 34
10 FAQ 35
10.1 Multichannel system setup .................................................. 35
10.1.1 Bang & Olufsen television as source ........................................... 35
10.1.2 Third-party device as source ............................................... 35
10.2 Does BeoLab 50 support DSD? ................................................ 35
10.3 Does BeoLab 50 support DXD? ................................................ 35
10.4 Why does the BeoLab 50 sound “different” when I switch to watching television? ..................... 35
11 Setup “Tips and Tricks” 36
12 Troubleshooting 37
12.1 Some features in the BeoLab 50 interface are disabled ................................... 37
12.2 Lip Sync problems ....................................................... 37
12.3 Echo problems ......................................................... 37
12.3.1 Multiroom audio systems ................................................. 37
12.3.2 Surround Processors .................................................... 37
12.4 Loudspeakers don’t turn on automatically .......................................... 37
12.5 Loudspeakers never shut off .................................................. 37
12.5.1 Analogue sources ..................................................... 37
12.5.2 Digital sources ....................................................... 37
12.5.2.1 S/P-DIF and Optical ............................................... 37
12.6 The BeoLab 50 interface doesn’t work ............................................ 37
12.7 Loudspeakers are distorting .................................................. 37
12.8 Loudspeakers are noisy / too quiet .............................................. 37
12.9 USB Audio not working ..................................................... 38
12.10 S/P-DIF input not working ................................................... 38
12.11 Optical input not working ................................................... 38
12.12 Automatic switching of inputs not behaving as expected .................................. 38
13 Appendix 1: Recommendations for Critical Listening 39
4
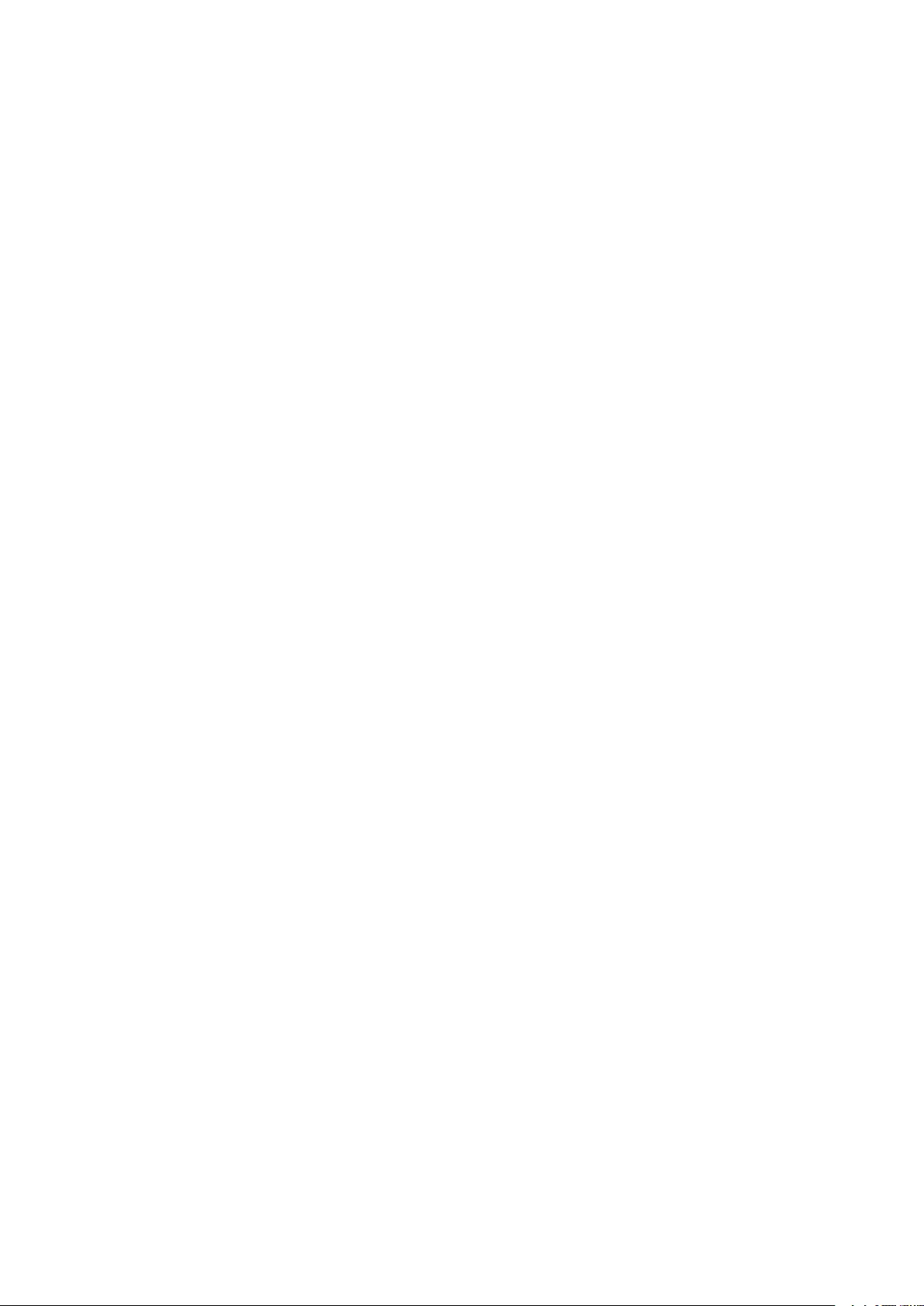
13.1 Loudspeaker Configuration .................................................. 39
13.2 Listening Room Acoustics ................................................... 40
13.3 Source Devices ......................................................... 40
13.4 Cable recommendations .................................................... 41
13.4.1 Analogue cables ...................................................... 41
13.4.2 Optical cables ....................................................... 41
13.4.3 S/P-DIF cables ....................................................... 41
13.5 AC mains cables ........................................................ 41
14 Appendix 2: Introduction to Parametric Equalisers 42
14.1 Filter Type ............................................................ 42
14.1.1 low-shelving Filter ..................................................... 42
14.1.2 high-shelving Filter .................................................... 42
14.1.3 Peaking Filter ........................................................ 43
14.2 Gain ............................................................... 43
14.3 Centre Frequency ........................................................ 43
14.4 Q ................................................................ 43
15 Appendix 3: The Influence of Listening Room Acoustics on Loudspeakers 45
15.1 Early Reflections ........................................................ 45
15.2 Room Modes .......................................................... 47
15.3 Reverberation ......................................................... 48
15.4 Solutions ............................................................ 48
15.5 Conclusions ........................................................... 48
16 Appendix 4: Loudspeaker Directivity and Distance Perception in Stereo Imaging 50
16.1 Distance Perception in Real Life ................................................ 50
16.2 Distance Perception in a Stereo Recording .......................................... 50
16.3 Combining the Two ....................................................... 50
17 Appendix 5: Microphone placement strategy when creating ARC Zones 51
17.1 General information ...................................................... 51
17.1.1 Background noise ..................................................... 51
17.1.2 Microphone Orientation and Holder ............................................ 51
17.1.3 Height ........................................................... 51
17.1.4 Doors and Windows .................................................... 51
17.2 One listening position ..................................................... 51
17.3 More than one listening position ................................................ 52
18 Appendix 6: ABL - Adaptive Bass Linearisation 53
18.1 A General Introduction to ABL ................................................. 53
18.2 ABL and BeoLab 50 ....................................................... 54
19 Appendix 7: Thermal Compression Compensation 55
19.1 Introduction ........................................................... 55
19.2 Voice coil temperature ..................................................... 55
19.3 Loudspeaker response changes ................................................ 56
5
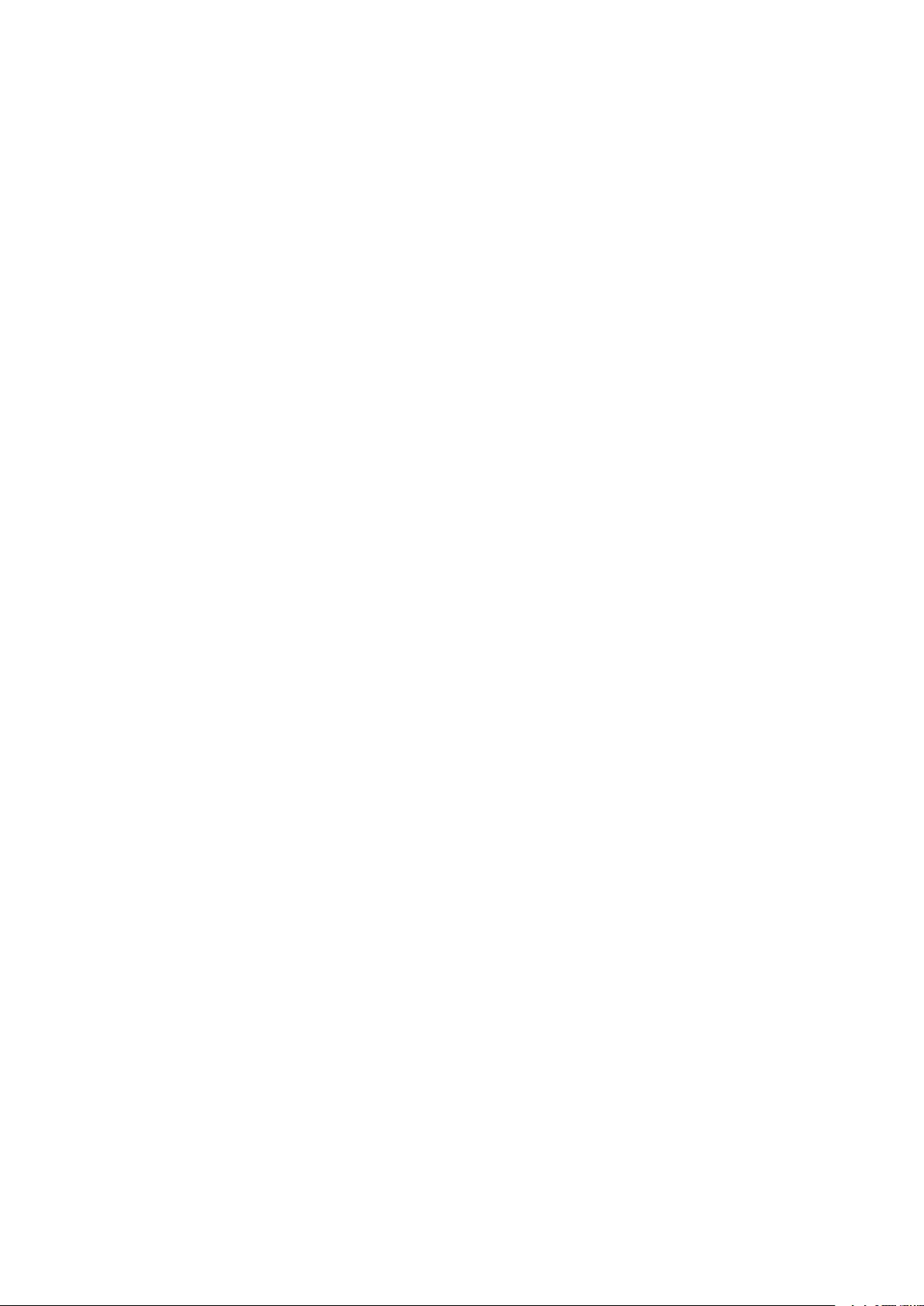
19.4 The solution .......................................................... 56
19.5 Some extra information .................................................... 57
20 Appendix 8: Control of BeoLab 50 using the BeoRemote 1 58
20.1 Introduction ........................................................... 58
20.1.1 Customising the Product Name .............................................. 58
20.2 Input Selection ......................................................... 58
20.2.1 Source Selection ...................................................... 58
20.2.2 Customising the Input Names ............................................... 58
Index 59
6
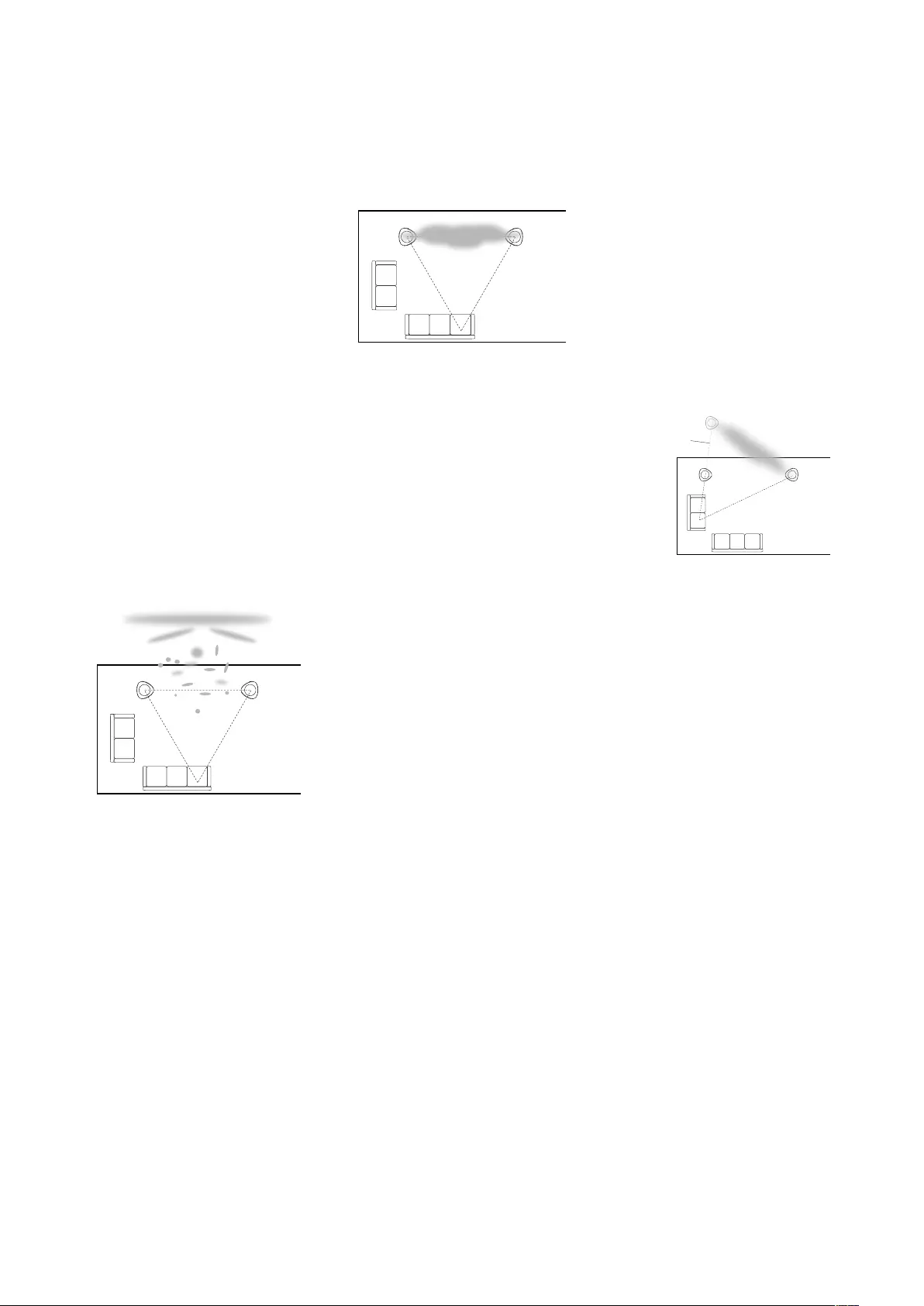
Introduction
The BeoLab 50 is a loudspeaker
concept from Bang & Olufsen that
gives the customer an unprecedented
level of control of its acoustic
behaviour and performance. Like the
flagship BeoLab 90, the BeoLab 50 can
be altered by the user to behave as if it
were completely different loudspeakers
for different listening situations.
Imagine that you have a pair of BeoLab
50 loudspeakers, perfectly positioned
in your listening room, with a single
chair in the correct location, as is
shown in Figure 1.1. You sit in this chair
to listen to a recording – to hear
sparkling high frequencies and a tight,
punchy bass that extends to the lowest
audible frequency bands along with
the accurate and precise placement of
the instruments within the space in
front of you (better known as “stereo
imaging”).
Speaker Distance
Compensation
Perceived location of
left loudspeaker
Figure 1.1: The sound stage presented
by BeoLab 50’s for active listening when
sitting in the sweet spot with properly
placed loudspeakers.
Now, imagine that you have the same
loudspeakers in the same positions in
the same room, but you’ve moved to
the sofa as in Figure 1.3 (or perhaps
you’re still in the same chair as before
as in Figure 1.2) and you prefer to hear
music in the background while you
read a book. In this case, the bass
precision and the imaging of the
recording is not important – you just
want a cloud of sound that does not
distract you while you read. Using your
controller you simply switch the
behaviour of the BeoLab 50’s to deliver
this experience instead.
Speaker Distance
Compensation
Perceived location of
left loudspeaker
Figure 1.2: The sound stage presented
by BeoLab 50’s for passive listening
when sitting in the sweet spot.
These two scenarios illustrate the
primary listening modes that the
BeoLab 50 can deliver. We’ll call the
first one active listening – since your
primary activity is to listen to the
recording. We’ll call the second one
passive listening, since, in this case,
listening to music is secondary to
another activity (in our example,
reading) in a stationary listening
position or area. In order to be able to
do this, there are many different
adjustable parameters inside the
BeoLab 50. You can choose to alter
each of these parameters individually
according to your preferences and
listening positions – and then save the
settings to a preset for future use.
1.1 Features
BeoLab 50 gives you the power to
make these changes using a large
number of “handles” – controllers that
let you change the acoustical
behaviour of the loudspeaker. Among
these features, there are two that
stand out:
•Beam Width Control
•Active Room Compensation
In addition to these, the BeoLab 50 has
a many other parameters that give you
a wide range of customisation
possibilities such as:
•Speaker Distance (for
time-alignment at the listening
position)
•Speaker Level
•Basic Tone Controls
(Bass, Treble, Frequency Tilt,
Sound Enhance)
•Selectable Sound Designs
•10-band Parametric Equaliser
Speaker Distance
Compensation
Perceived location of
left loudspeaker
Figure 1.3: The sound stage presented
by BeoLab 50’s for passive listening
when not sitting in the sweet spot.
1.1.1 Beam Width Control
When a recording engineer makes a
recording in a well-designed studio, he
or she is sitting not only in a
carefully-designed acoustical space,
but a very special area within that
space. In many recording studios,
there is an area behind the mixing
console where there are no reflections
from the sidewalls arriving just after
the direct sound from the
loudspeakers. This is accomplished
either by putting acoustically
absorptive materials on the walls to
soak up the sound so it cannot reflect
(as shown in Figure 1.4), or to angle
the walls so that the reflections are
directed away from the listening
position (as shown in Figure 1.5).
7
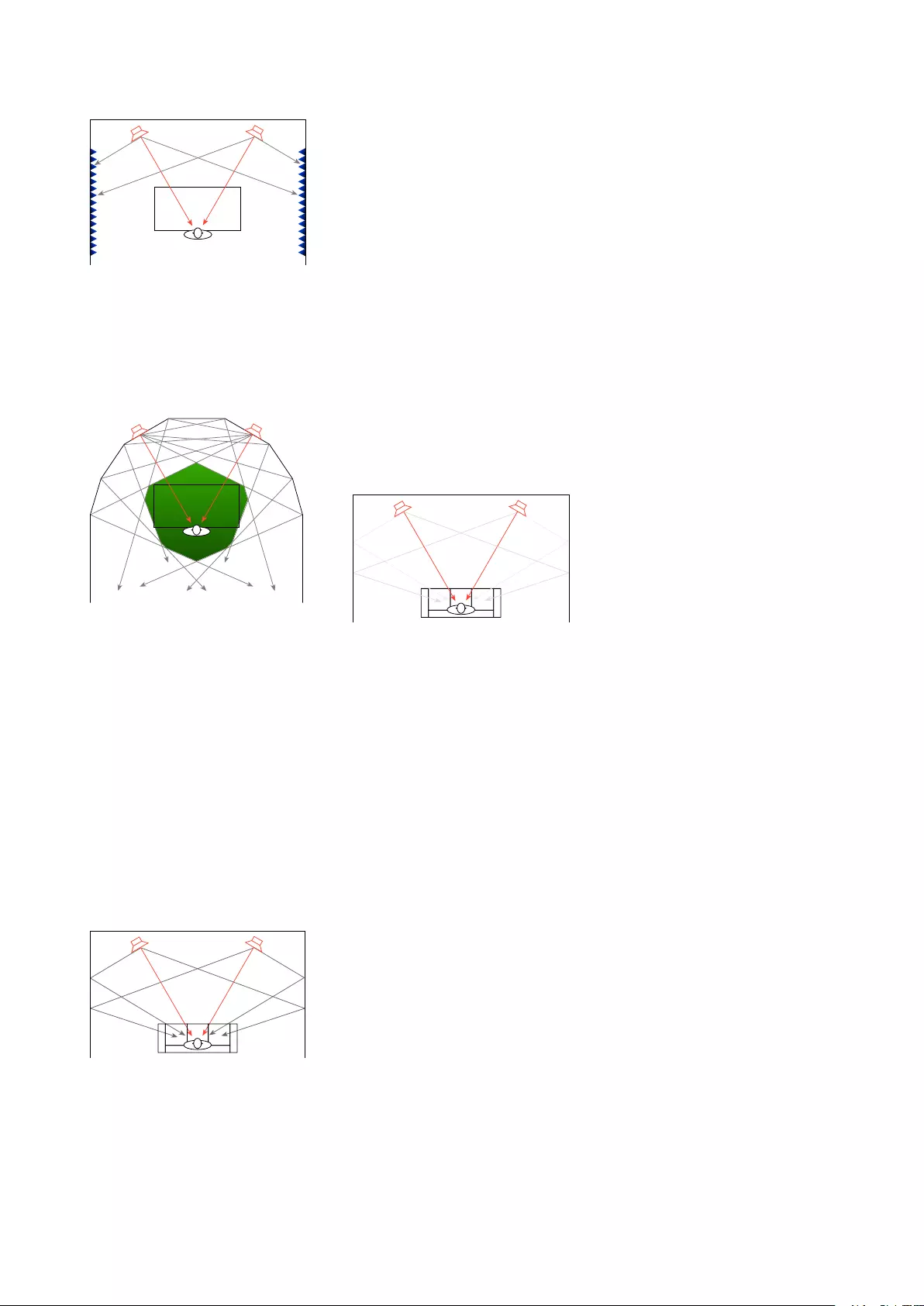
"Reflection-free Zone"
Figure 1.4: One way to reduce the prob-
lem of side wall reflections is to absorb
them at the walls so that there is no re-
flection. This is a solution often used in
recording studios, however, it also re-
sults in an unnatural-sounding “dead”
room.
"Reflection-free Zone"
Figure 1.5: An alternative method to re-
duce the problem of side wall reflections
is to re-direct them away from the lis-
tening position, producing a “reflection-
free zone”. This method is often used
in recording studios that are initially de-
signed with the help of an experienced
acoustical consultant.
This is different from your living room
which has not be designed primarily as
an acoustical space. It has sidewalls
that reflect the energy from your
loudspeakers and send that sound to
you at the listening position – a
situation that is more like that which is
shown in Figure 1.6.
"Reflection-free Zone"
Figure 1.6: The direct sound (in red)
from the loudspeakers is influenced by
the reflections off the side walls (in
grey).
In order to get the same acoustical
behaviour in your living room that the
recording engineer had, we have to
reduce the amount of energy that is
reflected off the side walls. If we do not
want to change the room, one way to
do this is to change the behaviour of
the loudspeaker by focusing the beam
of sound so that it stays directed at the
listening position, but it sends less
energy to the sides, towards the walls.
This is one of the options that BeoLab
50 gives you – to make the beam of
sound directed out the front of the
loudspeaker narrower to reduce the
level of sidewall reflections, so that you
get a more accurate representation of
the sound the recording engineer
heard when the recording was made.
"Reflection-free Zone"
Figure 1.7: BeoLab 50 solves the prob-
lem of side wall reflections by reduc-
ing the amount of acoustic energy that
is radiated towards the side walls – so
there is less energy to reflect.
However, if you’re sharing your music
with friends or family, depending on
where people are sitting, the beam
may be too narrow to ensure that
everyone has the same experience. In
this case, it may be desirable to make
BeoLab 50’s sound beam wider.
This option to change the pattern of
the radiation of sound from the BeoLab
50 is called Beam Width Control.
1.1.2 ARC: Active Room
Compensation
In 2002, Bang & Olufsen introduced
the BeoLab 5 which included ABC –
Automatic Bass Calibration. This was a
system that used a microphone to
measure the effects of the listening
room’s acoustical behaviour on the
sound of the loudspeaker, and then
created a filter that compensated for
those effects in the low frequency
band. As a simple example, if your
room tended to increase the apparent
bass level, then the BeoLab 5’s would
reduce their bass level by the same
amount.
BeoLab 90 took this concept to a new
level with its Active Room
Compensation or ARC, and that system
is also available in the BeoLab 50.
Using an external microphone
(supplied with the loudspeaker), you
can measure the effects of your room’s
acoustical behaviour in different zones
in the room and subsequently select
optimised compensation filters for
different situations. For example, you
can customise a filter for the sofa, and
another for your dining area. In cases
where you are moving between these
locations, you can simply select a
combination of both filters to create a
single compensation filter that
improves the sound experience in both
locations.
The BeoLab 50 also offers another
development in acoustical room
compensation: multichannel
processing. This means that the
loudspeakers not only “see” each other
as having an effect on the room – but
they help each other to control the
room’s acoustical influence.
8
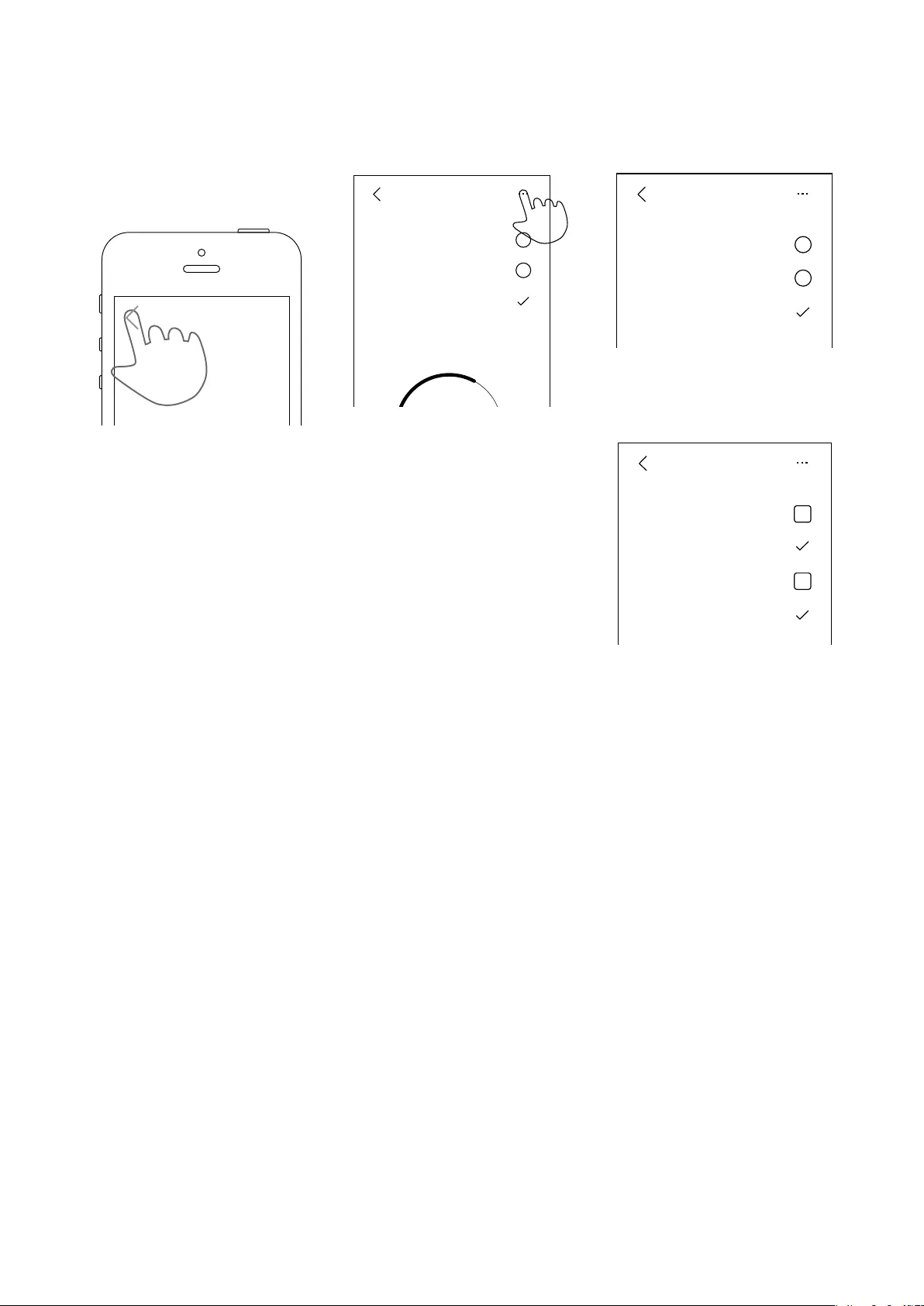
Application Information
2.1 Menu Navigation
Figure 2.1: Press the left-pointing arrow-
head at the top left of the screen to re-
turn to the previous menu.
Presets
Easy Chair
Party
Sofa Left
Presets
Easy Chair
Party
Sofa Left
Presets
Easy Chair
Party
Sofa Left
Presets
Easy Chair
Party
Sofa Left
+
Presets
Easy Chair
Party
Sofa Left
...
Presets
Easy Chair
Party
Sofa Left
...
Presets
Easy Chair
Party
Sofa Left
...
Figure 2.2: Press the “...” icon in the top
right to switch to the edit mode for the
current screen.
Presets
Easy Chair
Party
Sofa Left
Presets
Easy Chair
Party
Sofa Left
Presets
Easy Chair
Party
Sofa Left
Presets
Easy Chair
Party
Sofa Left
+
Presets
Easy Chair
Party
Sofa Left
...
Presets
Easy Chair
Party
Sofa Left
...
Presets
Easy Chair
Party
Sofa Left
...
Figure 2.3: Circular selection buttons al-
low for one item from the list to be cho-
sen.
Room Compensation
Sweet Spot
Sofas
Entire Room
...
Dining Table
Room Compensation
Sweet Spot
Sofas
Entire Room
...
Dining Table
Room Compensation
Sweet Spot
Sofas
Entire Room
Dining Table
+
Figure 2.4: Rounded square selection
buttons are toggles that allow for more
than item from the list to be chosen.
9
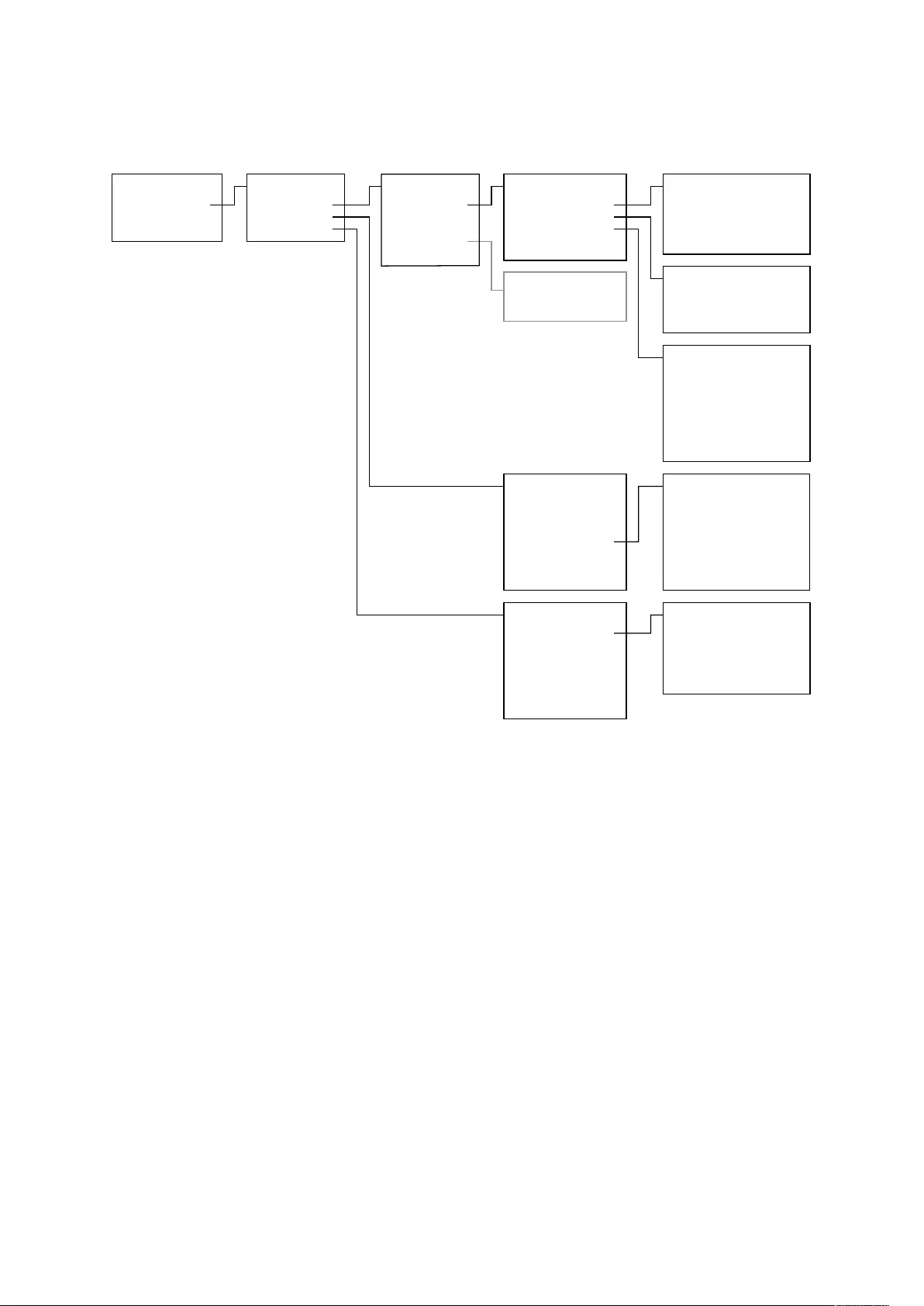
2.2 Menu Map
Beolab 50 Presets ...
PRESETNAME
PRESETNAME
Volume / Mute
Beam Control Beam Width
Latency Mode
Parametric EQ
Loudness
Frequency Tilt
Sound Enhance
Sound Designs
Preset Number
ZONENAME
ZONENAME
+
Speaker Role
Speaker Distance
Speaker Level
Room Compensation
Advanced
Rename
Tone Controls
+
PRESETNAME
Wireless PowerLink
Automatic
Power Link
RCA
S/P-DIF
Optical
USB Audio
Inputs ...
Bass
Treble
Tone Controls
Beam Control
Room Compensation ...
Gain Offset
Listening Preset
Detect Threshold
Max Input Voltage
Control Volume
Input Impedance
Time-Out
INPUTNAME
About
Guide
Network
Reset
Power Enhance
Max Volume
Default Volume
System
Automatic Update
UTC Time
Search for Update
Submit Log
Product information
About
Speaker
SPEAKERNAME Preset
Input
System
SPEAKERNAME
etc.
Advanced
Figure 2.5: Simplified and generalised navigational map for the BeoLab 50 interface when completed. Some items shown above are
only visible when the menu is in edit mode which is entered by pressing the “...” icon at the top right of some menus. Also, not all
parameters are available for all items (e.g. different inputs have different options).
10
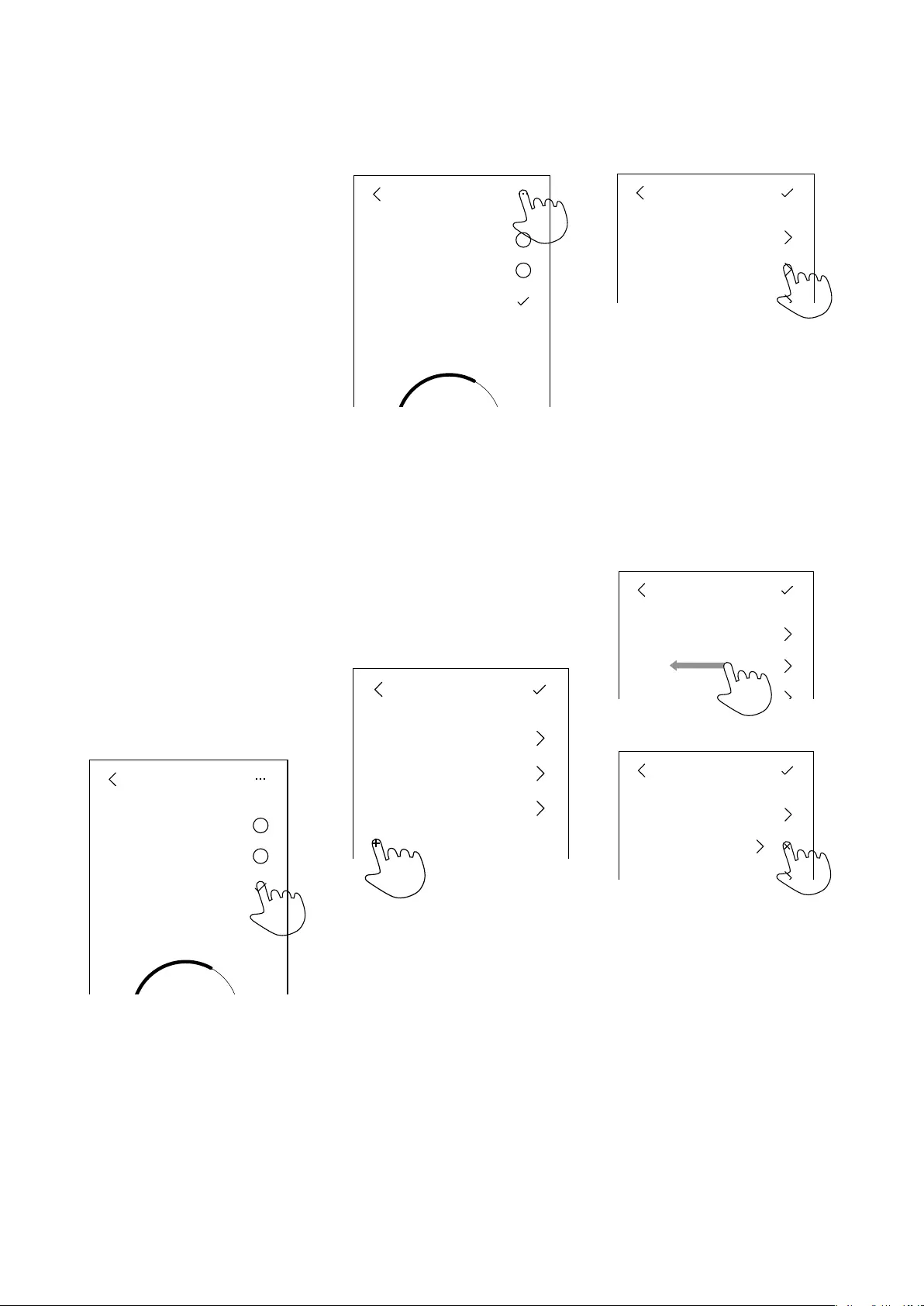
Presets
3.1 What is a Preset?
Almost all parameters that can affect
the audio characteristics of the BeoLab
50 can be pre-programmed and saved
as a preset that is easily and quickly
selectable by the end user. A preset
contains a wide range of controls that
can be customised to suit both the
listener’s personal preferences and his
or her location in the listening room.
Presets can either be selected
manually using the BeoLab 50
interface or they can be selected
automatically as is explained in
Automating Preset Selection.
3.2 Preset management
3.2.1 Selecting a Preset
The list of currently-available presets
are shown in the Preset Select menu,
an example of which is shown in Figure
3.1. From this menu, you can manually
select a preset by clicking on its icon
as shown, or you can move deeper into
the Edit Preset menu as shown in
Figure 3.2.
Presets
Easy Chair
Party
Sofa Left
Presets
Easy Chair
Party
Sofa Left
Presets
Easy Chair
Party
Sofa Left
Presets
Easy Chair
Party
Sofa Left
+
Presets
Easy Chair
Party
Sofa Left
...
Presets
Easy Chair
Party
Sofa Left
...
Presets
Easy Chair
Party
Sofa Left
...
Figure 3.1: Select a Preset by pressing
its icon on the right of the screen as
shown. The currently-selected Preset is
indicated with a check mark.
Presets
Easy Chair
Party
Sofa Left
Presets
Easy Chair
Party
Sofa Left
Presets
Easy Chair
Party
Sofa Left
Presets
Easy Chair
Party
Sofa Left
+
Presets
Easy Chair
Party
Sofa Left
...
Presets
Easy Chair
Party
Sofa Left
...
Presets
Easy Chair
Party
Sofa Left
...
Figure 3.2: Press the three dots at the
top right of the screen to enter the edit
mode.
3.2.2 Creating a Preset
In order to create a new preset, enter
the edit mode (as shown in Figure 3.2)
and press the “+” icon in the Preset
menu. This will start a process where
you can name the preset and edit its
parameters.
Presets
Easy Chair
Party
Sofa Left
Presets
Easy Chair
Party
Sofa Left
Presets
Easy Chair
Party
Sofa Left
Presets
Easy Chair
Party
Sofa Left
+
Presets
Easy Chair
Party
Sofa Left
...
Presets
Easy Chair
Party
Sofa Left
...
Presets
Easy Chair
Party
Sofa Left
...
Figure 3.3: Press the “+” icon to create
a new preset. Note the check mark on
the top right of the screen which indi-
cates that we have entered the “edit”
mode.
3.2.3 Editing a Preset
To edit the parameters of an existing
preset, press its associated icon after
you have entered the preset menu’s
edit mode (by pressing the ‘...’ icon on
the top right of the screen).
Presets
Easy Chair
Party
Sofa Left
Presets
Easy Chair
Party
Sofa Left
Presets
Easy Chair
Party
Sofa Left
Presets
Easy Chair
Party
Sofa Left
+
Presets
Easy Chair
Party
Sofa Left
...
Presets
Easy Chair
Party
Sofa Left
...
Presets
Easy Chair
Party
Sofa Left
...
Figure 3.4: Press anywhere on a preset’s
line to begin to edit its parameters.
3.2.4 Deleting a Preset
In order to delete a preset, enter the
preset menu’s edit mode and swipe to
the left at any position in the row. This
will reveal an “x” on the right side of
the screen. Pressing the “x” will delete
the preset.
Presets
Easy Chair
Party
Sofa Left
Presets
Easy Chair
Party
Sofa Left
Presets
Easy Chair
Party
Sofa Left
Presets
Easy Chair
Party
Sofa Left
+
Presets
Easy Chair
Party
Sofa Left
...
Presets
Easy Chair
Party
Sofa Left
...
Presets
Easy Chair
Party
Sofa Left
...
Figure 3.5: An example of deleting a
preset. To delete the “Party” preset,
swipe to the left on its row. This will re-
veal the “x” on the right of the screen.
Press the “x” to delete the preset.
11
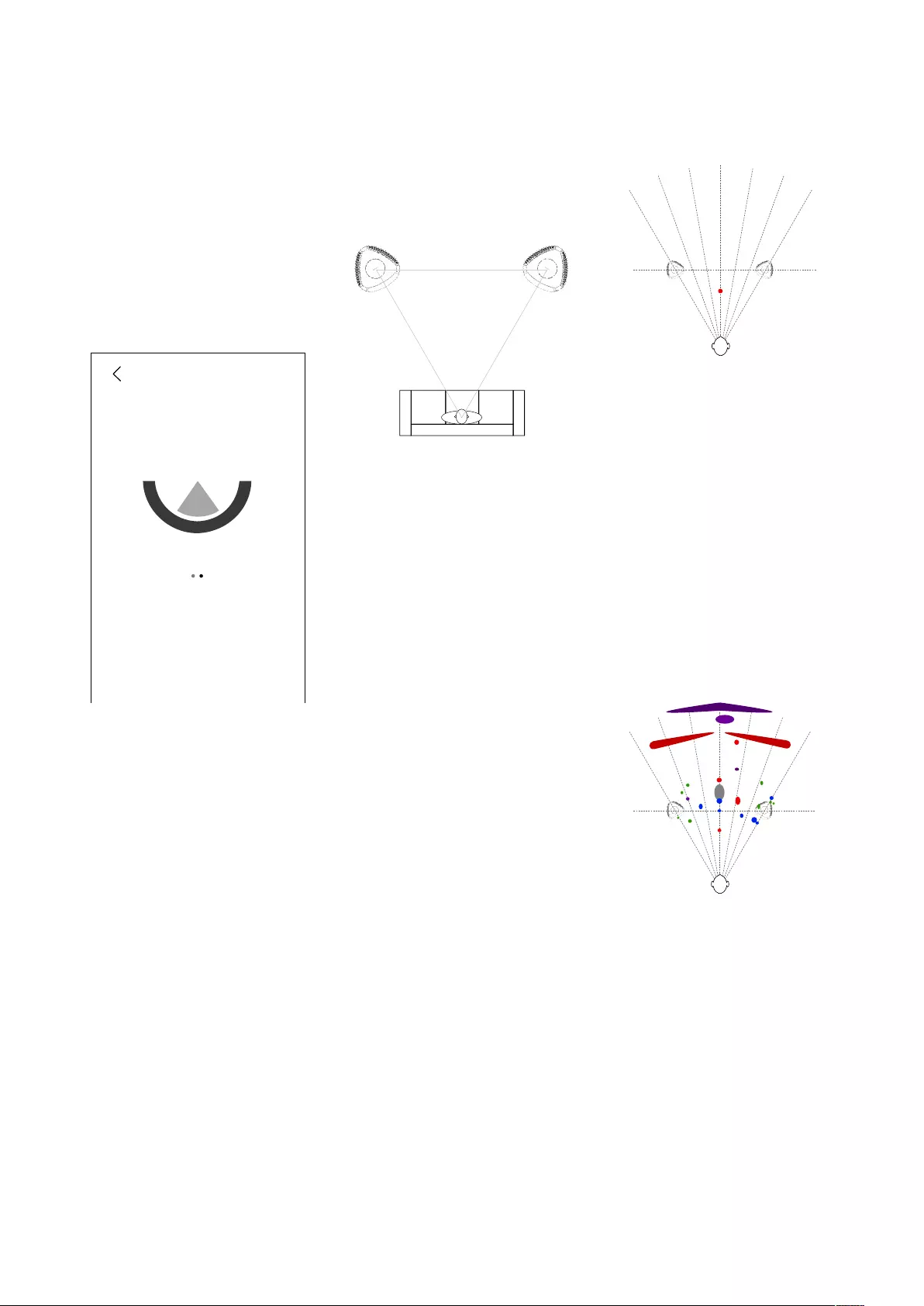
Control Parameters
4.1 Beam Width Control
The beam of sound that is radiated
from the BeoLab 50 can be adjusted by
selecting from two options:
•Narrow
•Wide
Beam Control
Master
Distance
Level
Left
3.5 m
5.0 dB
Master
Distance
Level
Left
3.5 m
5.0 dB
Master Left
3.5 m
5.0 dB
Wide sound
... Beam Control
Wide sound
... Beam Control
Level
Wide sound
Figure 4.1: The Beam Control menu for
the Master loudspeaker. To switch be-
tween the Master and the Slave loud-
speaker, swipe the upper area of the
screen left and right.
Note that, if an Active Room
Compensation zone has been created,
and is applied to the preset you are
currently editing, then an appropriate
filter setting will be calculated each
time you change Beam Width. This
calculation takes approximately 20
seconds to perform, and is indicated by
a progress wheel. If you wish to
audition differences in Beam Width
more quickly, this can be done by
disabling the ARC filter for the preset in
the Room Compensation menu.
4.1.1 Narrow
Sit in the “sweet spot” – a location in
your listening room that is exactly the
same distance from each of your
BeoLab 50’s, and where the two
loudspeakers are facing (shown in
Figure 4.2). Using your BeoLab 50
interface, set the Beam Width Control
to “Narrow”.
"Reflection-free Zone"
Figure 4.2: A “perfect” loudspeaker con-
figuration with BeoLab 50’s. Both loud-
speakers are aimed at the listening po-
sition. The distance from the listen-
ing position to each loudspeaker is the
same as the distance between the two
loudspeakers. For more detailed infor-
mation on loudspeaker configuration,
see Appendix 1: Recommendations for
Critical Listening
While facing a point located at the
centre between the two loudspeakers,
play “Tom’s Diner” (recorded by
Suzanne Vega in 1987 for her album
“Solitude Standing”). Vega’s voice
should appear to float at a position
between the two loudspeakers. If her
voice does not appear to be located
exactly mid-way between the two
loudspeakers, it is likely that you are
sitting slightly closer to one
loudspeaker than the other – in other
words, to one side of the sweet spot.
Try moving slightly side-to-side and
pay attention to the lateral movement
of Vega’s voice in space.
This ability for a pair of loudspeakers to
deliver the illusion of a sound coming
from a location in space between them
is called phantom imaging or stereo
imaging or simply imaging.
Now pay attention to the apparent
distance to the voice. If the Beam
Width Control of the loudspeakers is
set to “Narrow” mode, the voice will
appear to floating at a distance
somewhere between you and the
loudspeakers. This is illustrated in
Figure 4.3.
tri
tri
tom tom
tom
hh
voice
bongos
bk
bk
bass
bass
gtr
synth pad at end
sax
snare
"choir"
"choir"
cow
synth fx around 2:20
shake
tri
tri
Figure 4.3: A map of the phantom im-
age location of the voice (shown in red)
in Suzanne Vega’s recording of Tom’s
Diner. Beam Width: Narrow
Change the track to Jennifer Warnes’s
recording of “Bird on a Wire” from the
Album “Famous Blue Raincoat: The
Songs of Leonard Cohen”. In this
recording, there are many more
instruments and voices, however, it
should be very easy to locate the
position of each of those sources as
coming from somewhere between the
two loudspeakers. A partial map of
these locations is shown in Figure 4.4.
tri
tri
tom tom
tom
hh
voice
bongos
bk
bk
bass
bass
gtr
synth pad at end
sax
snare
"choir"
"choir"
cow
synth fx around 2:20
shake
tri
tri
Figure 4.4: A map of the phantom im-
age locations of instruments and voices
in Jennifer Warnes’s recording of Bird on
a Wire. Beam Width: Narrow.
BeoLab 50 is able to deliver such a
precise stereo imaging for active
listening because it is able to reduce
the amount of energy in the reflections
off the side walls of your listening
room. This gives the same result at the
listening position as if you used
acoustically absorptive materials on
12
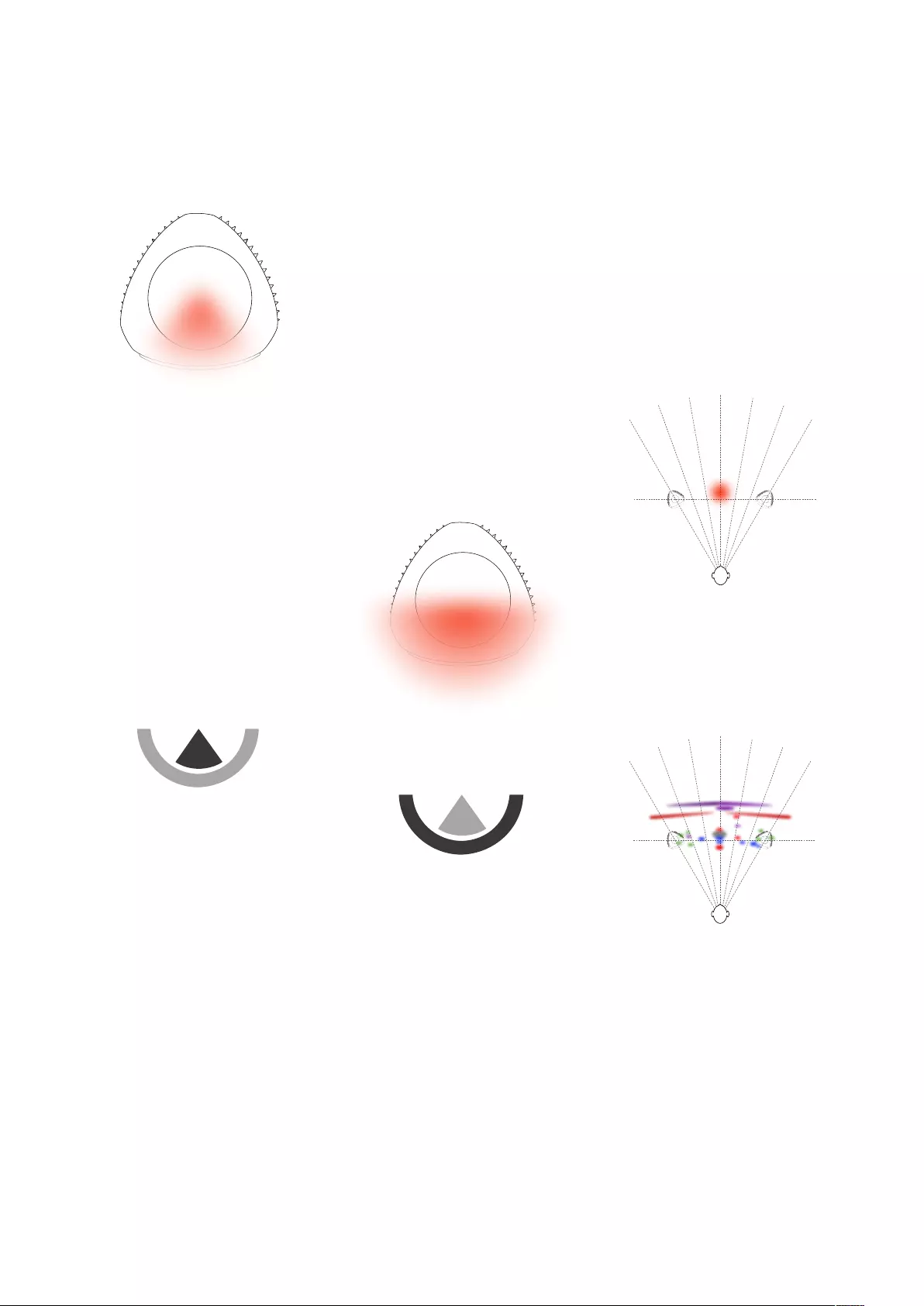
your walls, or changed the geometry of
your listening room to avoid having
first reflections in the listening position.
Figure 4.5: Conceptual drawing showing
the beam width of the Narrow Beam.
You should note, however, that there
are side-effects to using a narrow
beam width. The most obvious may be
in the low frequency behaviour of your
BeoLab 50’s. Generally, the overall
impression will be that the bass
content is “tighter” or has more
“punch” when the BeoLab 50 is in
narrow mode. However, this effect is
also dependent on the setting of
another parameter described in
Latency Mode.
Figure 4.6: Press the sector (or “pizza
slice”) on the BeoLab 50 interface to
change the Beam Width to Narrow.
A second potential side effect is the
sensitivity of the system to an incorrect
listening position. You may notice that,
in narrow mode, it is critical that you
are seated at exactly the correct
listening position in order to achieve
both precise and accurate stereo
imaging. Small deviations in listening
position may result in noticeable
detriments in the spatial
representation of your recordings.
4.1.2 Wide
As mentioned above, when the BeoLab
50’s are set to a narrow beam width,
they are somewhat unforgiving of a
mis-placement of the listening
position. This is particularly noticeable
when you are listening to recordings or
movies with friends and family.
Consequently, in more social or
passive listening situations, it is likely
preferable that the BeoLab 50’s have a
wider beam width, more similar to
BeoLab 5 loudspeakers. Although this
will likely result in more energy in the
sidewall reflections, it also ensures that
there is a more equal distribution of
the direct sound across a wider
listening area in the room.
Figure 4.7: Conceptual drawing showing
the beam width of the Wide Beam.
Figure 4.8: Press the curved line shown
above to change the Beam Width to
Wide (Front).
The side effects of the Wide beam
width are dependent on the strength of
the sidewall reflections, however, in
many situations, four different effects
may be audible.
The first is that the apparent distance
to the various sources in the stereo
mix will collapse slightly, resulting in
the perception that the sources in the
recording are roughly the same
distance from the listening position as
the loudspeakers themselves. This
means that (relative to the narrow
mode) very close sources will move
further away and very far sources will
move closer to the listening position.
Secondly, the apparent width of the
sources will become slightly larger with
less precise left-right locations. You will
not have pinpoint locations as in
narrow mode – imaging becomes
slightly more “cloudy” or “fuzzy”. This
is due to the extra energy reflected off
the side walls.
tri
tri
tom tom
tom
hh
voice
bongos
bk
bk
bass
bass
gtr
synth pad at end
sax
snare
"choir"
"choir"
cow
synth fx around 2:20
shake
tri
tri
Figure 4.9: A map of the phantom im-
age location of the voice (shown in red)
in Suzanne Vega’s recording of Tom’s
Diner. Beam Width: Wide. Compare to
Figure 4.3
tri
tri
tom tom
tom
hh
voice
bongos
bk
bk
bass
bass
gtr
synth pad at end
sax
snare
"choir"
"choir"
cow
synth fx around 2:20
shake
tri
tri
Figure 4.10: A map of the phantom im-
age locations of instruments and voices
in Jennifer Warnes’s recording of Bird on
a Wire. Beam Width: Wide. Compare to
Figure 4.4
Thirdly, the overall timbre or tone
colour of the sound may change as a
result of increase influence of the
sidewall reflections at the listening
position.
13
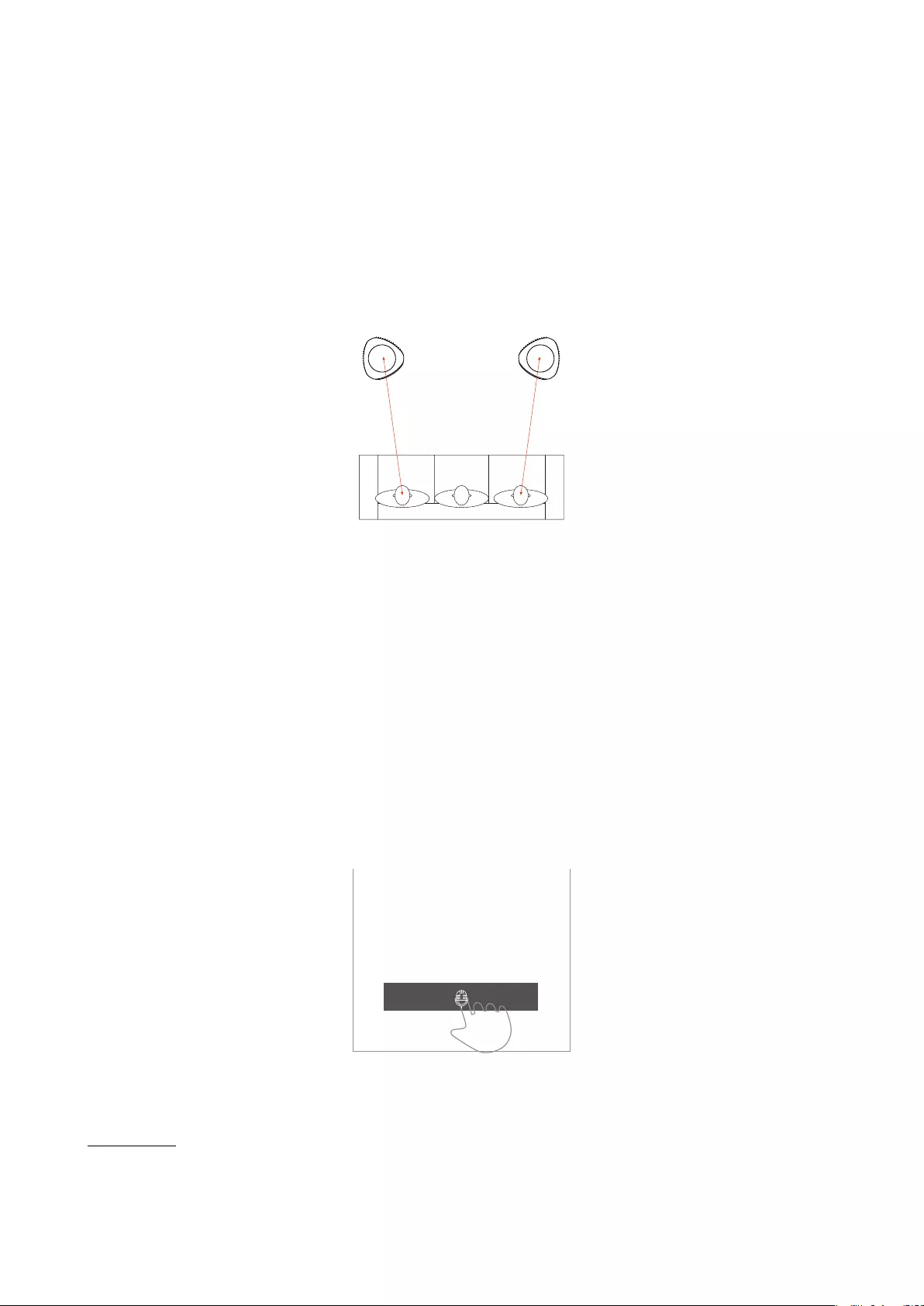
Finally, as mentioned above, the
overall “punch” of the bass will change
when compared to the narrow mode.
4.1.3 Comment
Note that the above illustrations
connecting Beam Widths to listener
position are merely that – illustrations.
It should also be said that changing the
Beam Width of the BeoLab 50 has
non-intuitive consequences on the
perceived sound of the loudspeakers.
For example, the overall sensation of
“punch” in the bass may be different
for the three Beam Widths, regardless
of your location in the listening room.
Consequently, it may be that you
prefer the overall sound of a particular
Beam Width, even if you are not sitting
“in the beam”.
4.2 Speaker Distance
The Speaker Distance control is used to
ensure that the times of arrival of the
loudspeakers’ signals at the listening
position are matched, despite their
being placed at different distances
from the listening position. The value
displayed on the menu should be the
distance from the listening position to
each loudspeaker. The result of this
alignment is that the closer
loudspeaker’s signal is delayed to
match the time of arrival of the sound
from the more distant loudspeaker.
Note that, since the Listening Position
can be different for different Presets,
these distances may not necessarily be
the same from Preset to Preset.
Units Metres, Feet
Range (m) 0.0 – 10.0
Range (ft) 0.0 – 30.0
Resolution 0.1
Factory Default 1.0 m
4.2.1 Adjusting Speaker
Distances for more than
one listening position
In cases where there is more than one
listener present, the Speaker Distances
can be optimised by measuring each
loudspeaker’s position relative to the
closest listening position, as is shown
in Figure 4.12.
Figure 4.12: The Speaker Distance for
each loudspeaker should be measured
from the relevant tweeter for the given
Beam Directions to the closest listening
position.
Automated Measurement of
Speaker Distance
The distance from the listening position
to each BeoLab 50 can be measured
automatically using the microphone
included with the loudspeakers. This is
done by pressing the microphone icon
at the bottom of the Beam Control
menu (shown in Figure 4.13) and
following the instructions.
Beam Control
Master
Distance
Level
Left
3.5 m
dB
5.0
180 directional sound
... Beam Control
Master
Distance
Level
Left
3.5 m
dB
5.0
180 directional sound
... Beam Control
Master
Level
Left
3.5 m
dB
5.0
180 directional sound
Figure 4.13: Pressing the microphone
icon in the Beam Control menu starts
the Speaker Distance measurement
procedure.
4.3 Speaker Level
The sensitivity of any two BeoLab 50’s
has been calibrated during their
creation to be within 1 dB of each other
at any third-octave frequency band
within their frequency range.1
However, there are cases where, due
to placement in the listening room,
room acoustics, or the listening
position relative to the loudspeakers,
you may wish to fine-tune the relative
levels of the two loudspeakers. This
can be done with the Speaker Level
adjustment.
It is recommended that the Speaker
Levels should be adjusted at the
listening position. Note that this can be
performed either before or after an
Active Room Compensation profile has
been created – the ARC compensates
for any adjustments automatically.
The Speaker Level for each BeoLab 50
in the pair is adjusted from the Beam
Control menu, shown in Figure 4.1.
4.4 Speaker Role
The BeoLab 50 is created as a pair of
loudspeakers – one “master”
loudspeaker which has the connection
panel for the input signals and one
“slave” loudspeaker.
Since both the left and right audio
channels are input to your master
loudspeaker, there is no physical way
of knowing which loudspeaker is on the
left and which is on the right (compare
Figures 4.14 and 4.15 as an example).
As a result, the interface allows you to
swap the Speaker Role, to ensure that
the correct audio channel is
reproduced by the correct loudspeaker.
The selection of Left or Right for the
Master and Slave loudspeakers is done
in the Beam Control menu, shown in
Figure 4.1.
1Preliminary specification. Subject to change.
14
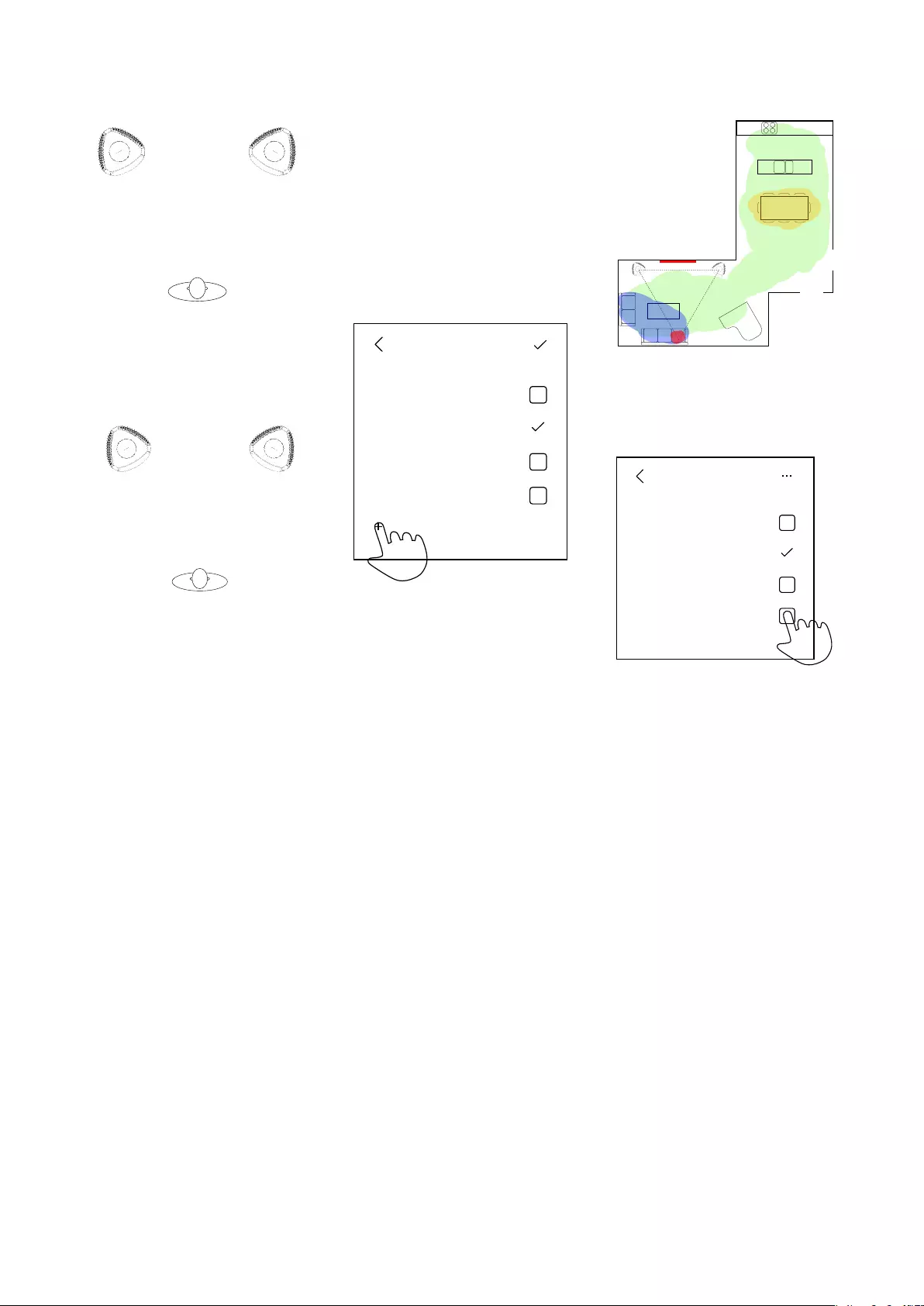
Master
(Left)
Slave
(Right)
Slave
(Left)
Master
(Right)
Master
(Right)
Slave
(Left)
Slave
(Right)
Master
(Left)
Figure 4.14: An example of a loud-
speaker configuration where the Mas-
ter loudspeaker should be assigned the
Speaker Role of “right”.
Master
(Left)
Slave
(Right)
Slave
(Left)
Master
(Right)
Master
(Right)
Slave
(Left)
Slave
(Right)
Master
(Left)
Figure 4.15: An example of a loud-
speaker configuration where the Mas-
ter loudspeaker should be assigned the
Speaker Role of “left”.
Note that a Master / Slave pair of
BeoLab 50’s cannot share the same
Speaker Role. If you wish to send the
same audio signal out of both
loudspeakers, this will have to be
arranged using the source device.
4.5 Active Room
Compensation
For a general introduction to the
effects of room acoustics on the sound
of a loudspeaker, please read Appendix
3: The Influence of Listening Room
Acoustics on Loudspeakers
It should be noted that the acoustical
behaviour of a room can change
considerably when windows or doors
are opened and closed. Consequently,
for optimal tuning, it is recommended
that ARC profiles be made for these
cases, particularly if this change is
made often (e.g. patio doors).
4.5.1 Creating a new ARC
Zone
A new Active Room Compensation
zone can be created by pressing the
“+” icon in the Room Compensation
Edit menu. (Enter the Room
Compensation Edit menu by pressing
the three dots at the top right of the
Room Compensation Select menu.)
Room Compensation
Sweet Spot
Sofas
Entire Room
...
Dining Table
Room Compensation
Sweet Spot
Sofas
Entire Room
...
Dining Table
Room Compensation
Sweet Spot
Sofas
Entire Room
Dining Table
+
Figure 4.16: Press the “+” icon in the
Room Compensation Edit menu to cre-
ate a new ARC zone.
This will start a procedure where you
will be guided through the process of
positioning the microphone in different
locations to optimised the ARC filters.
Each ARC is created using
measurements made in three
microphone locations, and a maximum
of 5 different ARC Zones can be
created.
For additional guidance, please see
Appendix 5: Microphone placement
strategy when creating ARC Zones.
4.5.2 Selecting an ARC Zone
It is possible to create up to 5 different
Active Room Compensation Zones that
can be recalled either manually, or
automatically as part of a Preset.
In order to disable the active room
compensation filters, simply de-select
them in the menu.
Figure 4.17: An example of a listening
space showing four different overlap-
ping ARC zones in red, blue, green, and
yellow.
Room Compensation
Sweet Spot
Sofas
Entire Room
...
Dining Table
Room Compensation
Sweet Spot
Sofas
Entire Room
...
Dining Table
Room Compensation
Sweet Spot
Sofas
Entire Room
Dining Table
+
Figure 4.18: Selecting one or more Ac-
tive Room Compensation zones accord-
ing to your listening area(s) in the room.
Note that it is possible to select more
than one zone simultaneously.
4.5.3 Combining ARC Zones
Up to three ARC Zones can be selected
simultaneously to create a filter that
incorporates the measurements from
the applicable areas of the listening
room.
Note, however, that adding an extra
zone to a current one may compromise
the quality of the audio signal in the
original zone. For example, if you have
two ARC Zones, one for the “Sweet
Spot” and the other for the “Dining
Table”, adding the Dining Table zone to
the Sweet Spot zone will reduce the
quality of the ARC filtering in the sweet
spot location. This is due to the fact
that some of the filtering required to
compensate for the room’s acoustical
15
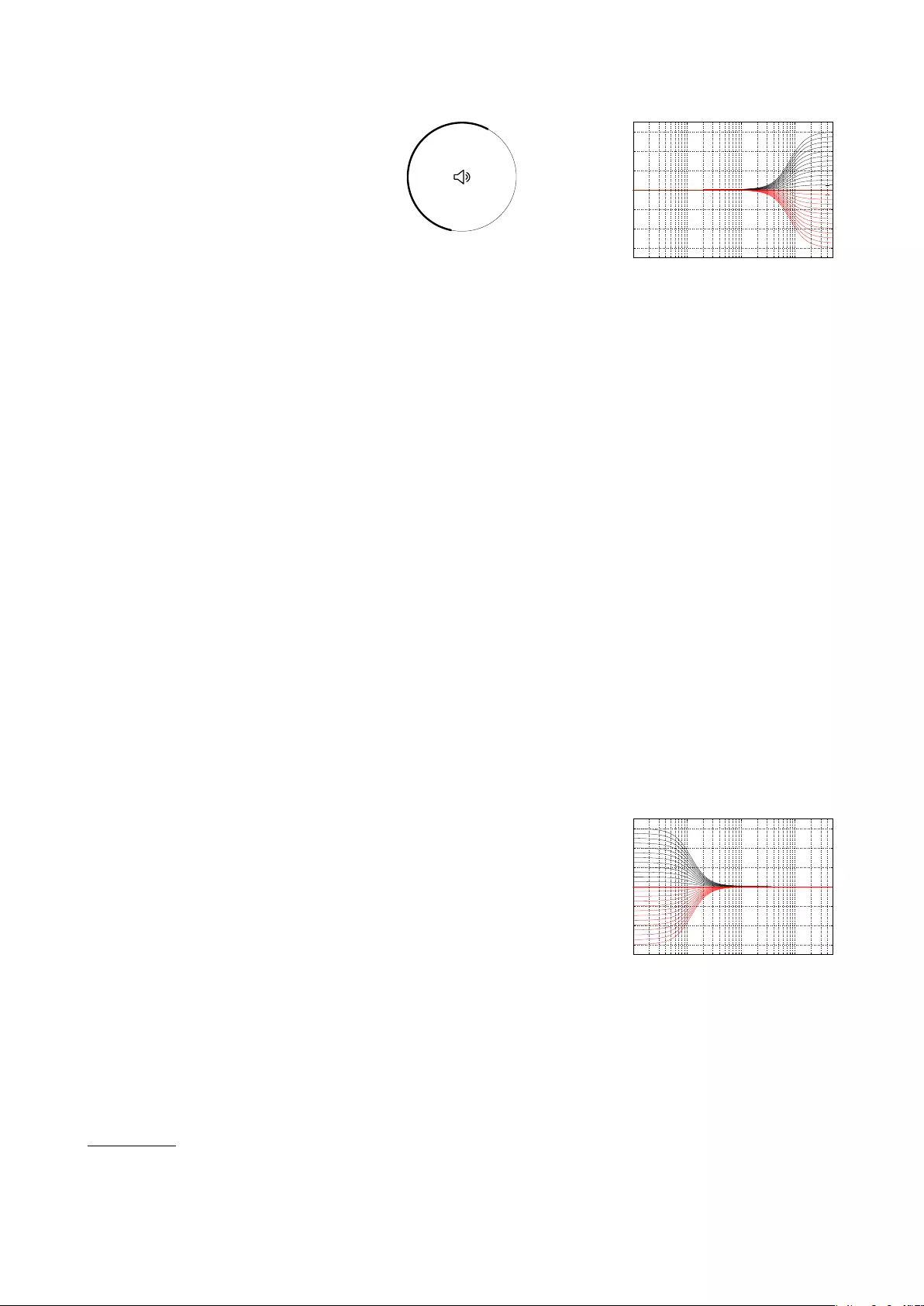
effects in the dining area may not be
required in the sweet spot.
Also note that changing Room
Compensation zones will cause an
approximately 20-second break in the
audio signal as the BeoLab 50
calculates and updates the appropriate
filters. This is normal.
4.6 Volume
The volume of the BeoLab 50 is
controllable from 0 to 90 in steps of 1
dB. Note that Volume Step 0 is a full
mute.
In its default settings, BeoLab 50 has
been calibrated to match the level of
other Bang & Olufsen loudspeakers for
its Power Link and Wireless Power Link
inputs. Tables 7.1 and 7.3 show the
output level of the loudspeaker for
various inputs and parameters.
Note that, although the Volume control
of the BeoLab 50 is disabled for Power
Link and Wireless Power Link sources,2
the volume of the source is duplicated
on the BeoLab 50 app. This is to
ensure that changes to a different
source are matched.
For example, say you have a BeoVision
Avant connected to Power Link input
and a CD player connected to the
S/P-DIF input of the BeoLab 50. You
start by listening to a CD at a high
volume level, then switch to watching
the television news at a low level (set
on the television). When you switch
back to listening to CD, the volume of
the BeoLab 50 will automatically have
been changed to the low setting of the
television.
4.7 Mute
Pressing the mute button in the centre
of the volume wheel reduces the
volume to a fixed value of 0.
Figure 4.19: The Volume control (the ex-
terior circle) and the Mute control (the
icon in the centre of the circle).
In order to unmute the sound, either
press the mute button again, or adjust
the volume.
Note that, if the volume setting of the
BeoLab 50 was higher than the startup
volume when muted, then the volume
setting after unmuting will be the same
as the startup volume.
4.8 Tone Controls
The Tone Controls on the BeoLab 50
consist of traditional Bass and Treble
controls. These are global adjustments
that are applied to all Presets and to
both loudspeakers simultaneously.
4.8.1 Treble
The Treble adjustment allows you to
change the relative amount of
high-frequency sound globally using a
high-shelving filter with a fixed
turnover frequency of 8 kHz and a Q of
0.707. Note that the gain at the
turnover frequency is one half the
maximum gain applied by the filter in
decibels. For example, when the gain
of the controller is -4 dB, the gain at 8
kHz is -2 dB.
The Treble control is applied to a global
filter and therefore is applied to all
Presets. It is also is independent of the
settings of other equalisation
controllers in the system such as the
Frequency Tilt, Sound Enhance and
Parametric Equaliser controls. The
range of the controller is from -6.0 dB
to +6.0 dB in steps of 0.5 dB.
10 100 1,000 10,000
−6
−4
−2
0
2
4
6
Frequency (Hz)
Gain (dB)
Figure 4.20: Magnitude Responses, Tre-
ble controller. Note that this filter is ap-
plied to both loudspeakers simultane-
ously.
4.8.2 Bass
The Bass adjustment allows you to
change the relative amount of
low-frequency sound globally using a
low-shelving filter with a fixed turnover
frequency of 120 Hz and a Q of 0.707.
The gain at the turnover frequency is
one half the maximum change in gain
applied by the filter in decibels. For
example, when the gain of the
controller is +6 dB, the gain at 120 Hz
is +3 dB.
The Bass control is a global filter and
therefore is applied to all Presets. It is
also is independent of the settings of
other equalisation controllers in the
system such as the Frequency Tilt,
Sound Enhance and Parametric
Equaliser controls. The range of the
controller is from -6.0 dB to +6.0 dB in
steps of 0.5 dB.
10 100 1,000 10,000
−6
−4
−2
0
2
4
6
Frequency (Hz)
Gain (dB)
Figure 4.21: Magnitude Responses:
Bass controller. Note that this filter is
applied to both loudspeakers simultane-
ously.
2This restriction is made to prevent incorrect calibration of levels in surround sound configurations.
16
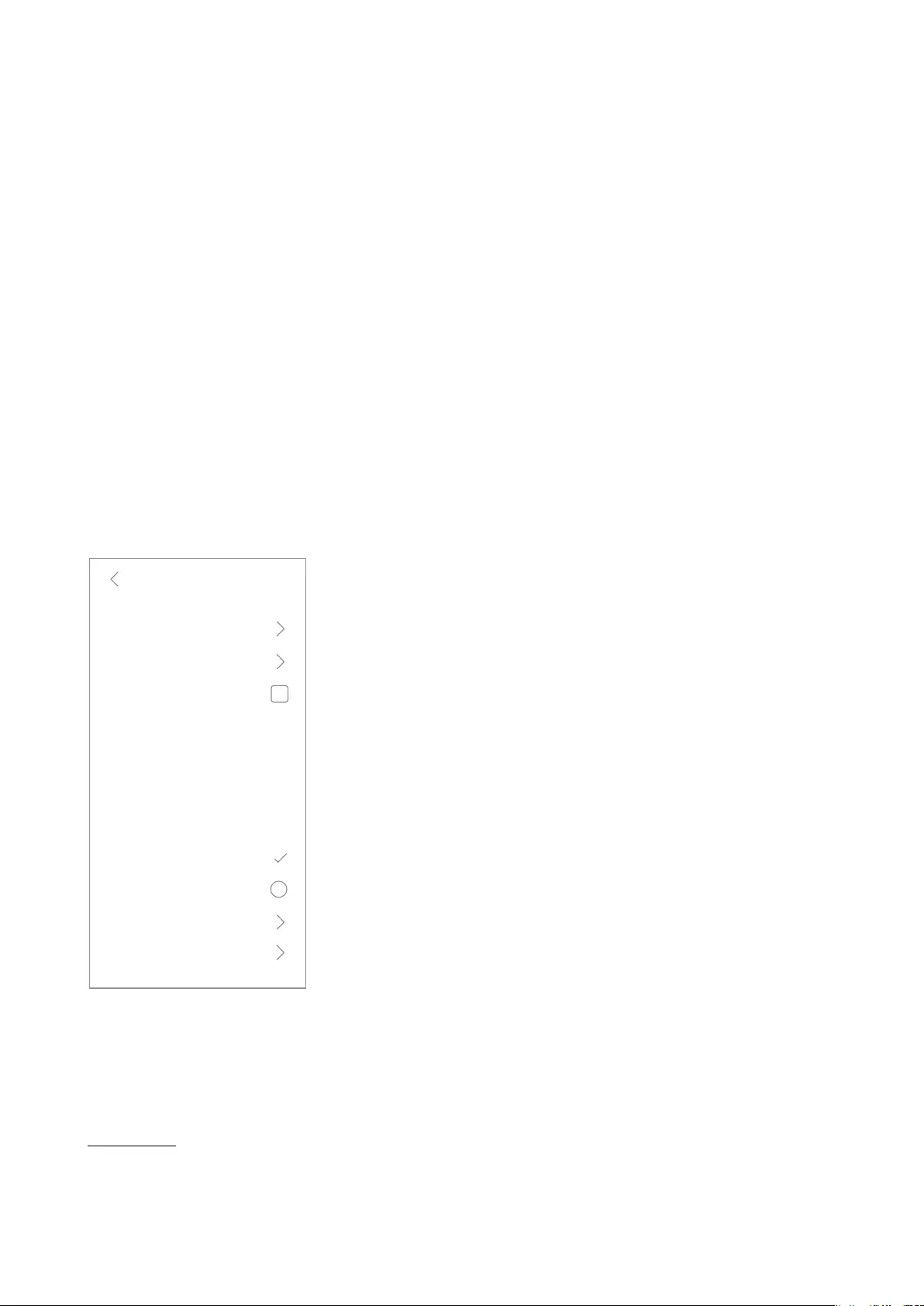
4.9 Advanced Controls
The Advanced Controls section gives
the user an almost-surgical control
over the timbral characteristics of the
BeoLab 50 using a combination of
legacy Bang & Olufsen audio
processing, standard equalisation tools
found in professional studio
equipment, and proprietary processing
available only in this loudspeaker.
The Advanced Controls of the BeoLab
50 are
•Latency Mode
•Loudness
•Frequency Tilt
•Sound Enhance
•Sound Design
•Parametric Equaliser
Advanced
Latency Mode High
Flat
0
0
Parametric EQ
Loudness
Frequency Tilt
Sound Enhance
Sound Design
Default
Flat on-axis
Trigger
Preset#
Figure 4.22: The Advanced Controls
menu.
4.9.1 Latency Mode
In order to control the Beam Width of
the sound radiating from the BeoLab
50, a customised Finite Impulse
Response (FIR) audio filter is selected
for each woofer and midrange to
accompany the changes resulting from
the moving Acoustic Lens above the
tweeter. These filters are applied to
each of the DSP’s 6 audio output
channels for those drivers. However, in
order to control the very low frequency
bands, it is necessary for the woofers’
FIR filters to be very long. One
implication of this is that it takes some
time between the moment an audio
signal enters the input of the
loudspeaker and the moment it exits
the loudspeaker as sound. The lower in
frequency the Beam Width Control is
extended, the longer the latency (or
delay) of the loudspeaker.
This ultimately means that there is a
direct relationship between the overall
latency of the loudspeaker and its
sound characteristics – especially in
the low frequency bands. One example
of this effect is: the longer the latency,
the “tighter” the bass.
However, this may mean that, for
some sources and program materials,
there is a loss of synchronisation. For
example, in its longest latency setting,
the loudspeaker may be too late to
maintain lip synch with some
televisions or some multiroom
systems. This is why the latency of the
loudspeaker is user-selectable between
two different settings.
Auto
If you are using BeoLab 50’s with a
current BeoVision television3, then the
Latency Mode should be set to “Auto”.
This will allow the television to manage
the latency mode of the loudspeakers
automatically.
Note that, if the Latency Mode is set to
“Auto” and the input is neither Power
Link nor Wireless Power Link, then the
BeoLab 50 will default to a High
Latency Mode of 100 ms.
High
To achieve the highest possible level of
audio quality from the BeoLab 50, the
internal digital processing must take
1/10th of a second in order to control
the low-frequency behaviour of the
system. This is selected by setting the
Latency Mode to “High”, thus
delivering the ultimate possible sound
quality from the loudspeaker.
However, there are cases where such a
long delay in the loudspeaker will
result in loss of synchronisation with
other devices in the system such as
the video (lip synch) or other
loudspeakers in a surround system. If
you are experiencing such problems,
then the lower latency mode should be
selected.
The latency of the BeoLab 50 in “High”
latency mode measured using an
analogue input is 100 ms.
Low
In some cases, a BeoLab 50 is
connected to a system that requires a
lower latency. One example of this is a
case where the loudspeaker is
connected to a non-B&O television or
surround processor. A second example
is a non-B&O multiroom system that
lacks the ability to adapt to different
loudspeaker latencies throughout the
network. Another example would be a
multichannel loudspeaker setup with a
non-B&O surround processor and a
mixture of different loudspeakers in the
configuration.
In this case, the overall delay of the
BeoLab 50 should be set to “Low” to
ensure synchronisation with other
loudspeakers in the system.
The latency of the BeoLab 50 in “Low”
latency mode measured using an
analogue input is 25 ms.
3BeoPlay V1, BeoVision 11, Avant, Avant NG, 14, Horizon, Eclipse – or later
17
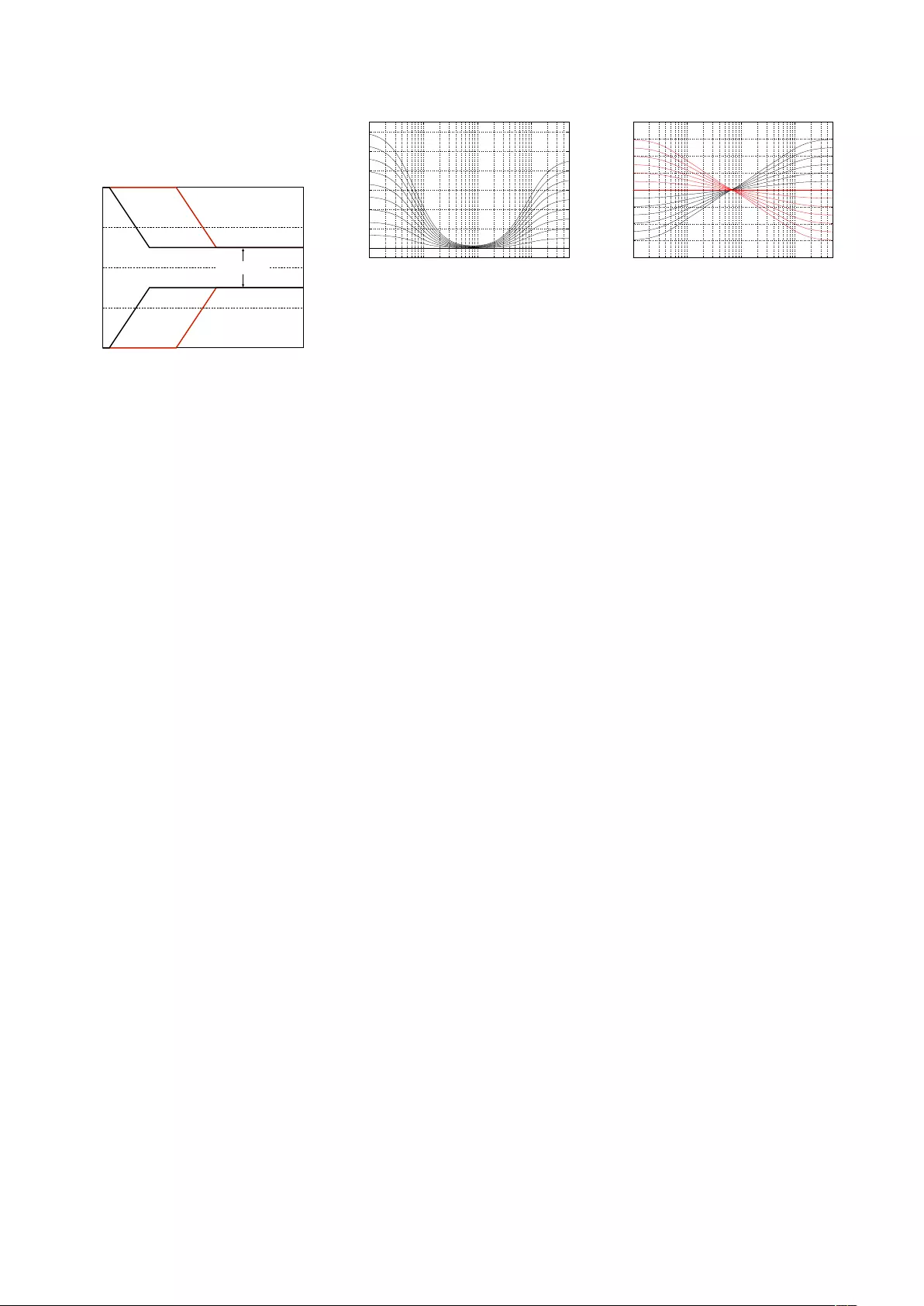
Effects of Latency Mode on
Beam Width
Frequency
Beam Width
FrontSide Side BackBack
Figure 4.23: Conceptual plot showing
the relationship between Latency Mode
and a Narrow Beam Width over fre-
quency. The black curve shows a High
latency mode. The red curve shows a
low latency mode. Note that the high
frequency beam width is the same for
both latency modes. Only the beam
width of the low frequency bands widen
for lower latencies.
4.9.2 Loudness
Sadly, human hearing is imperfect.
One of the issues that we all suffer
from is that our perception of the
timbre or “tone colour” of a sound is
not constant with listening level. We
are less sensitive to low frequencies
when they are played at low listening
levels. In other words, if you are
listening to music at a high level and
you turn down the volume, you will
notice that, the lower the volume, the
less bass you can hear. This is also true
of high frequencies, albeit to a lesser
extent.
The Loudness setting in your BeoLab
50’s counteracts this effect. As you
reduce the volume, the bass and treble
levels are automatically increased to
compensate for your reduced
perception in the outer frequency
bands.
If you do not wish this setting enabled,
Loudness should be set to OFF.
Note that the Loudness toggle
(whether it is on or off) is stored with
the Preset, so different modes can
have different settings.
Options On / Off
Default On
10 100 1,000 10,000
0
2
4
6
8
10
12
Frequency (Hz)
Gain (dB)
Figure 4.24: Magnitude responses of the
loudness function at various settings of
the volume control.
Note that, when connected to most
Bang & Olufsen sources, the Loudness
function in the BeoLab 50 will be
disabled for the Power Link and
Wireless Power Link inputs. This is
because in these cases, the Loudness
function is performed by the source
rather than the loudspeaker.
4.9.3 Frequency Tilt
Frequency Tilt can be considered to be
a combination of Bass and Treble
settings in a single parameter. When
Frequency Tilt is set to a low value, the
low frequency content of your audio
signal is increased and the level of the
high frequency content is reduced.
If the Frequency Tilt is set to a high
value, then the opposite will be true.
The Frequency Tilt function will have
no effect on the audio signal at its
middle setting.
Note that Frequency Tilt can have
different settings for different Presets.
The range of the controller is from -6.0
dB to +6.0 dB in steps of 0.5 dB. As
can be seen in Figure 4.25, a controller
setting of +6.0 will result in a
peak-to-peak magnitude response
deviation of approximately 6 dB,
however the maximum deviation from
a flat response is only 3 dB.
10 100 1,000 10,000
−4
−3
−2
−1
0
1
2
3
4
Frequency (Hz)
Gain (dB)
Figure 4.25: Magnitude Responses, Fre-
quency Tilt controller. Note that this fil-
ter is applied to both loudspeakers si-
multaneously. Black curves show the re-
sult for positive slider values, red curves
show negative slider values.
4.9.4 Sound Enhance
The Sound Enhance setting is similar to
the Frequency Tilt setting in that it
affects the low and high frequency
bands with a single slider. Increasing
the Sound Enhance value will increase
the level of the bass and treble bands
while reducing the midrange.
Decreasing the Sound Enhance value
will have the opposite effect and will
enhance the midrange.
The Sound Enhance setting will have
no effect on this audio signal at its
middle setting.
Note that Sound Enhance can have
different settings for different Presets.
The range of the controller is from -6.0
dB to +6.0 dB in steps of 0.5 dB. As
can be seen in Figure 4.26, a controller
setting of +6.0 will result in a
peak-to-peak magnitude response
deviation of approximately 6 dB,
however the maximum deviation from
a flat response is only 3 dB.
18
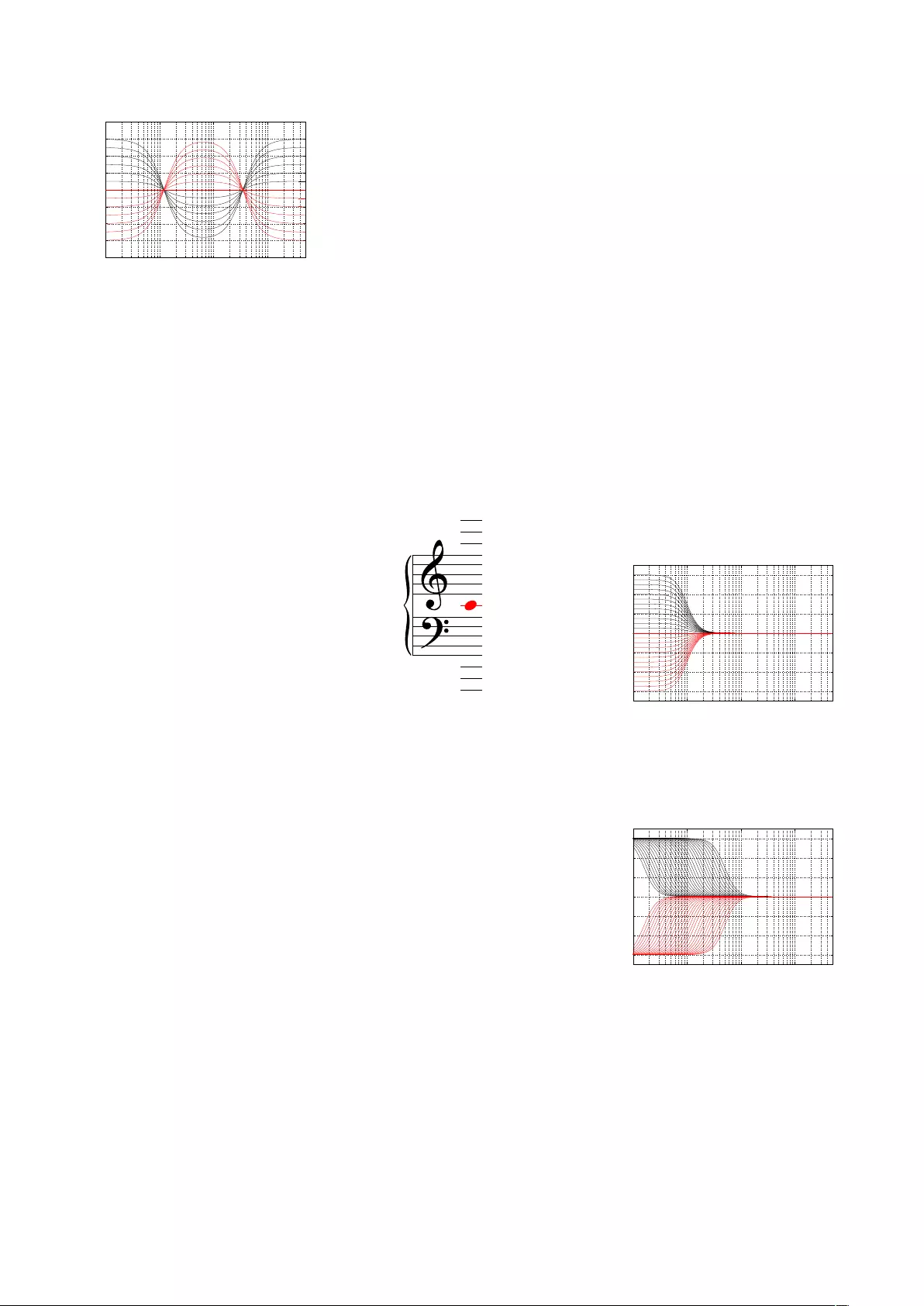
10 100 1,000 10,000
−4
−3
−2
−1
0
1
2
3
4
Frequency (Hz)
Gain (dB)
Figure 4.26: Magnitude Responses,
Sound Enhance controller. Note that
this filter is applied to both loudspeak-
ers simultaneously. Black curves show
the result for positive slider values, red
curves show negative slider values.
4.9.5 Sound Design
At the end of the development process,
all Bang & Olufsen loudspeakers go
through a final tuning process where
the loudspeaker’s timbre is evaluated
in different listening environments. In
order to achieve an optimised balance
between the on-axis frequency
response and the three-dimensional
“power response”, filters are included
in the signal path to give the
loudspeaker a final sound design.
The BeoLab 50 is no exception to this –
as a result, it has a custom-tuned,
factory-default sound design for every
combination of beam widths, beam
directions, and latency modes.
However, there may be some specific
cases where this tuning is not
applicable. One example of this is a
case where the BeoLab 50 is used in a
listening room such as a recording
studio where acoustical absorption has
been applied to the various surfaces.
In this case, it may be preferable to
use the BeoLab 50 as a “studio
monitor” style of loudspeaker, where
the overall tuning is designed to
deliver a flat magnitude response
when measured on-axis to the
loudspeaker in a free field.
The Sound Design control allows you to
switch between these two tunings. It is
currently planned that additional sound
designs will be made available in
future software releases.
4.9.6 Parametric Equaliser
For a general introduction to
equalisation, please see Appendix 2:
Introduction to Parametric Equalisers.
In cases where a more detailed control
of the frequency response of the
loudspeaker is needed, a 10-band
parametric equaliser is available. This
allows you to sculpt the timbral
balance of the loudspeaker with a high
degree of precision.
When the gains of all ten filters in the
Parametric Equaliser are set to 0 dB,
the processing block is automatically
disabled.
Figure 4.27 is given as a rough “map”
of frequency as reference when using
the Parametric Equaliser.
440.0 Hz
660.0 Hz
880.0 Hz
1320.0 Hz
220.0 Hz
261.6 Hz
330.0 Hz
110.0 Hz
165.0 Hz
55.0 Hz
55.0 Hz
Figure 4.27: Pitch vs. Fundamental
frequency for reference purposes when
equalising.
BeoLab 50’s Parametric EQ consists of
one low-shelving filter, one
high-shelving filter, and 8 reciprocal
peak-dip (or peaking) filters with
different frequency ranges as listed in
Table 7.4. Each filter has a variable
Frequency, Gain, and Q.
The centre frequencies of all filters
have ranges limited to 5 octaves in
order to optimise their signal-to-noise
ratios while providing a wide range of
control. Frequencies are limited to ISO
1/6th octave centres as listed in Table
7.5.
The available Q’s of the filters are
limited to the values listed in Table 7.6.
Note that all filters are implemented in
series, and that frequencies may
overlap each other in cases where
additional gain is desired.
All filters in the Parametric EQ section
are implemented as minimum phase
filters.
In order to ensure phase matching of
the two loudspeakers and therefore to
maintain phantom imaging
characteristics, identical Parametric
Equaliser parameters are applied to
both loudspeakers simultaneously.
Magnitude Response Plots
Low-Shelving Filter
The BeoLab 50 Parametric Equaliser
has one low-shelving filter available
with a frequency range of 16.0 Hz to
500.0 Hz and a Q range of 0.35 to 1.
The gain ranges from -6.0 dB to +6.0
dB in steps of 0.5 dB.
10 100 1,000 10,000
−6
−4
−2
0
2
4
6
Frequency (Hz)
Gain (dB)
Figure 4.28: Magnitude Responses, Ad-
vanced EQ, low-shelving filter: Fc = 100
Hz, Gain varied from -6.0 to +6.0 dB, Q
= 1.
10 100 1,000 10,000
−6
−4
−2
0
2
4
6
Frequency (Hz)
Gain (dB)
Figure 4.29: Magnitude Responses, Ad-
vanced EQ, low-shelving filter: Fc varied
from 16 to 500 Hz., Gain = ±6 dB, Q =
1.
19
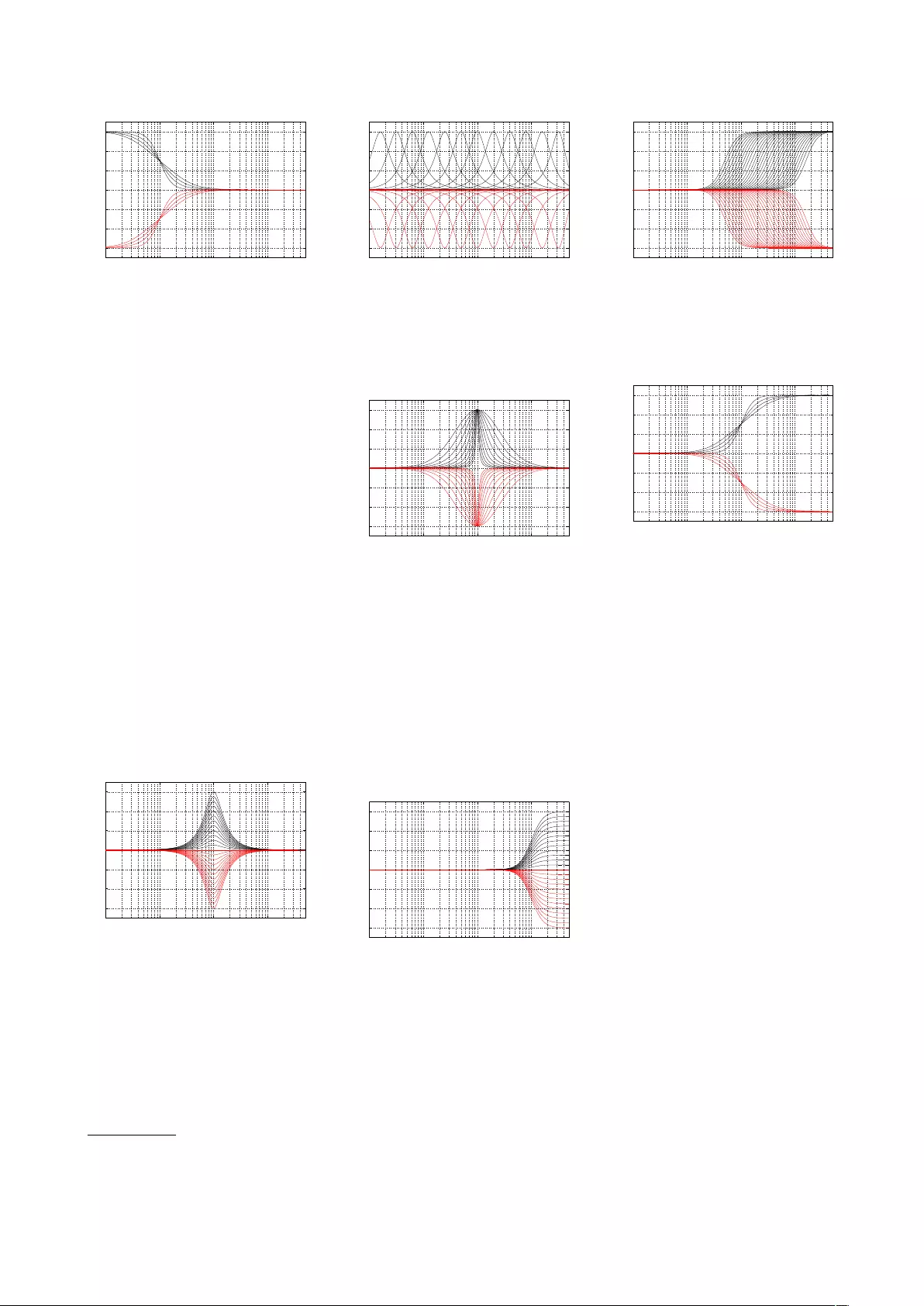
10 100 1,000 10,000
−6
−4
−2
0
2
4
6
Frequency (Hz)
Gain (dB)
Figure 4.30: Magnitude Responses, Ad-
vanced EQ, low-shelving filter: Fc = 100
Hz, Gain = ±6 dB, Q varied from 0.35 to
1.
Peaking Filters
The BeoLab 50 Parametric Equaliser
has eight reciprocal peak-dip or
peaking filters available. All peaking
filters have a Q value that ranges from
0.35 to 8.0 where the Q is based on a
bandwidth defined by the half-gain
points4. The gain ranges from -6.0 dB
to +6.0 dB in steps of 0.5 dB. The
peaking filters have a range of 5
octaves with differing limits as follows:
•Four low-frequency filters with a
range of 16.0 Hz to 500.0 Hz.
•Three mid-frequency filters with
a range of 250.0 Hz to 8.0 kHz.
•One high-frequency filter with a
range of 2.0 kHz to 63.0 kHz.
10 100 1,000 10,000
−6
−4
−2
0
2
4
6
Frequency (Hz)
Gain (dB)
Figure 4.31: Magnitude Responses, Ad-
vanced EQ, Peaking filter: Fc = 100 Hz,
Gain varied from -6.0 to +6.0 dB, Q = 1.
10 100 1,000 10,000
−6
−4
−2
0
2
4
6
Frequency (Hz)
Gain (dB)
Figure 4.32: Magnitude Responses, Ad-
vanced EQ, Peaking filter: Examples of
Fc varied from 16 Hz to 32 kHz on one-
octave centres, Gain = ±6 dB, Q = 1.
10 100 1,000 10,000
−6
−4
−2
0
2
4
6
Frequency (Hz)
Gain (dB)
Figure 4.33: Magnitude Responses, Ad-
vanced EQ, Peaking filters: Fc = 100 Hz,
Gain = ±6 dB, Q varied from 0.35 to 8.
high-shelving Filter
The BeoLab 50 Parametric Equaliser
has one high-shelving filter available
with a frequency range of 500.0 Hz to
16.0 kHz and a Q range of 0.35 to 1.
The gain ranges from -6.0 dB to +6.0
dB in steps of 0.5 dB.
10 100 1,000 10,000
−6
−4
−2
0
2
4
6
Frequency (Hz)
Gain (dB)
Figure 4.34: Magnitude Responses, Ad-
vanced EQ, high-shelving filter: Fc =
1000 Hz, Gain varied from -6.0 to +6.0
dB, Q = 1. Note that this filter is applied
to both loudspeakers simultaneously.
10 100 1,000 10,000
−6
−4
−2
0
2
4
6
Frequency (Hz)
Gain (dB)
Figure 4.35: Magnitude Responses, Ad-
vanced EQ, high-shelving filter: Fc var-
ied from 500Hz to 16 kHz, Gain = ±6
dB, Q = 1.
10 100 1,000 10,000
−6
−4
−2
0
2
4
6
Frequency (Hz)
Gain (dB)
Figure 4.36: Magnitude Responses, Ad-
vanced EQ, high-shelving filter: Fc =
100 Hz, Gain = ±6 dB, Q varied from
0.35 to 1.
4.10 Automating Preset
Selection
It is not necessary to manually select
Presets using the BeoLab 50 app. It is
possible, instead, to have Presets
triggered to be selected automatically
using one of two possible external
controls: By Speaker Group (if you
have a Bang & Olufsen television such
as a BeoVision 11 or BeoVision Avant)
or By Source.
By Speaker Group
If you have a pair of BeoLab 50’s
connected to a Bang & Olufsen
television such as a BeoVision 11 or
BeoVision Avant as shown in Figure
4.37, then it is possible to
automatically trigger presets in
tandem with the television’s Speaker
Group. This selection is done in the
Speaker Group menus on the
television, where you can select the
4For more information on this, please see “The Equivalence of Various Methods of Computing Biquad Coefficients for Audio Parametric Equalizers” Robert Bristow-Johnson,
Preprint 3906, 97th International Convention of the Audio Engineering Society, November 1994
20
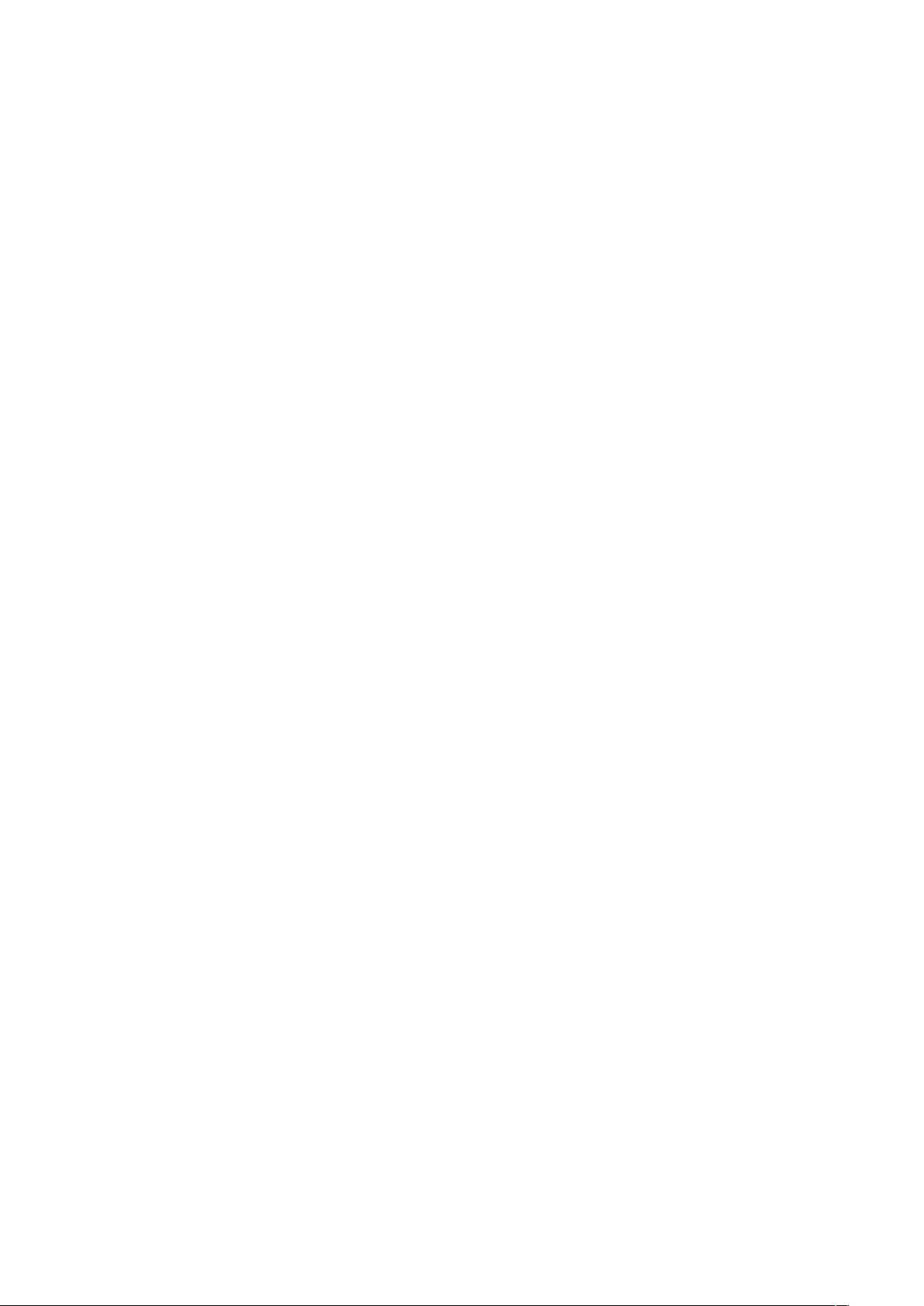
“Speaker Preset” number for the
BeoLab 50 as one of the parameters in
the Speaker Group. See the BeoVision
Technical Audio Guide for more
information about this.
Note that, in cases where a
multichannel loudspeaker
configuration includes more than one
pair of BeoLab 50’s or BeoLab 90’s
connected to a BeoVision television, it
will be necessary to ensure that the
Preset numbers are the same for all
pairs of loudspeakers in the system,
since the television sends out only one
Speaker Preset number for all
loudspeakers connected to it.
By Source
Imagine you have a pair of BeoLab
50’s connected to two non-B&O
sources as shown in Figure 4.38.
•an AV Surround Processor
connected to the RCA Line
inputs. The device is also
connected to other loudspeakers
to form a multichannel
(surround) configuration for
watching movies.
•a high-resolution audio player
connected to the S/P-DIF input.
In addition, you have configured two
Presets in your BeoLab 50’s:
1. Optimised for multichannel
listening with a listening zone
that encompasses more than one
listening position (e.g. the whole
sofa).
2. Optimised for 2.0 Stereo listening
with only one “sweet spot” in the
centre of the sofa.
In this situation, you want the AV
Surround processor to automatically
select Preset 1 and the high-resolution
audio player to automatically select
Preset 2. In this way, there is no need
to manually change BeoLab 50 presets.
Mixed systems
Note that is possible to trigger both by
source and by Speaker Group in mixed
systems such as that shown in Figure
4.39. In this case, the BeoVision
television is controlling the BeoLab 50
preset within its Speaker Group
parameters. However, the BeoLab 50
can also have a preset that is
automatically triggered by the audio
player connected via S/P-DIF.
21
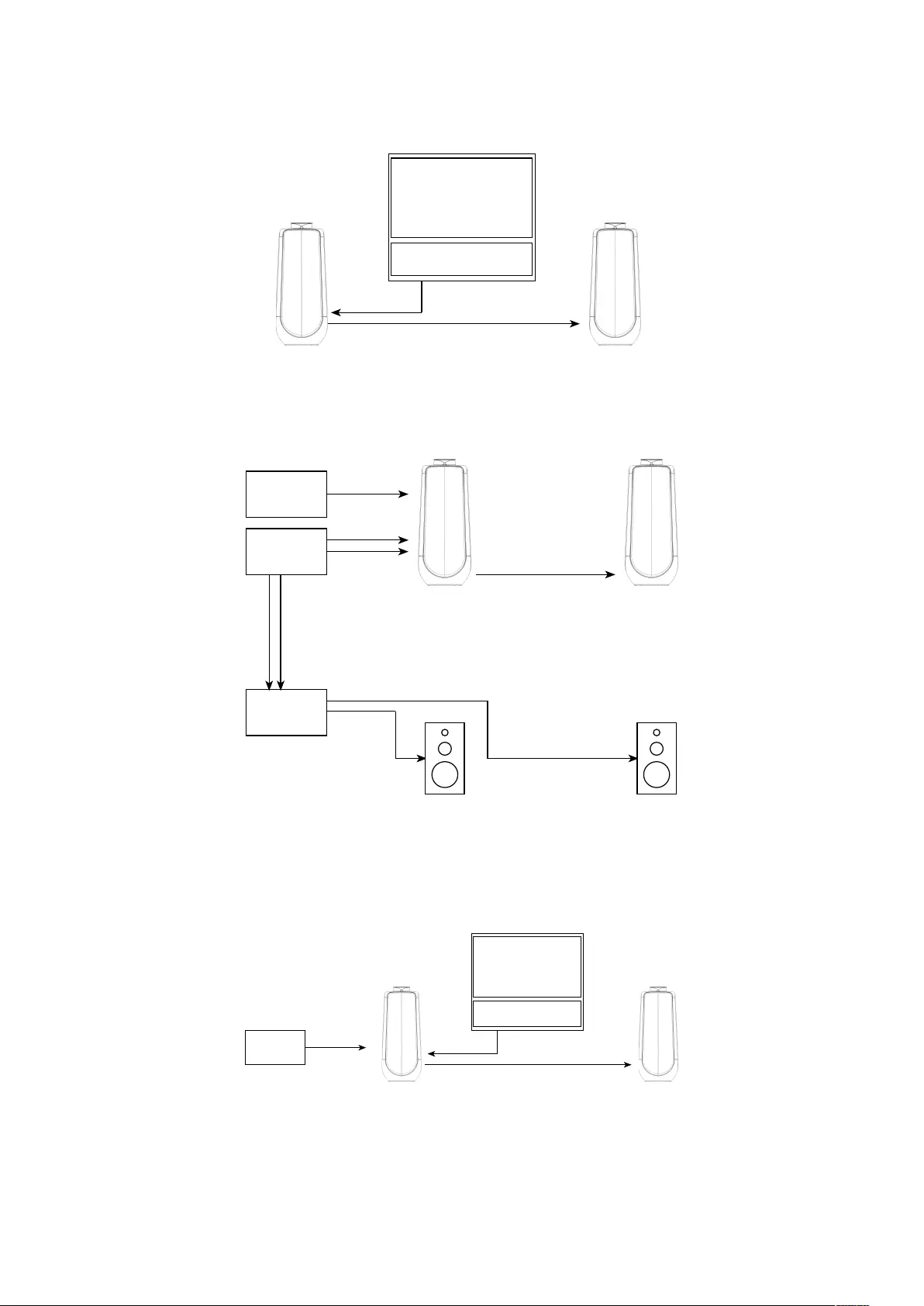
Audio Player
AV Surround
Processor
Power Amplifier
XLR
S/P-DIF
Audio Player S/P-DIF
Digital Power Link
Digital Power Link
Power Link
Digital Power Link
Power Link
Figure 4.37: An example of a pair of BeoLab 50’s connected to a BeoVision 11 using Power Link.
Audio Player
AV Surround
Processor
Power Amplifier
RCA
S/P-DIF
Audio Player S/P-DIF
Digital Power Link
Digital Power Link
Power Link
Digital Power Link
Power Link
Figure 4.38: An example of a pair of BeoLab 50’s connected to two third-party sources: an AV Surround Processor using RCA and a
separate audio player using S/P-DIF. Note that, in this case, the latency of the BeoLab 50’s must be carefully managed in the setup of
the loudspeakers and the AV Surround Processor in order to ensure that the multichannel system is behaving correctly.
Audio Player
AV Surround
Processor
Power Amplifier
XLR
S/P-DIF
Audio Player S/P-DIF
Digital Power Link
Digital Power Link
Power Link
Digital Power Link
Power Link
Figure 4.39: An example of a pair of BeoLab 50’s connected to one B&O source using Power Link and a third-party audio player using
S/P-DIF.
22
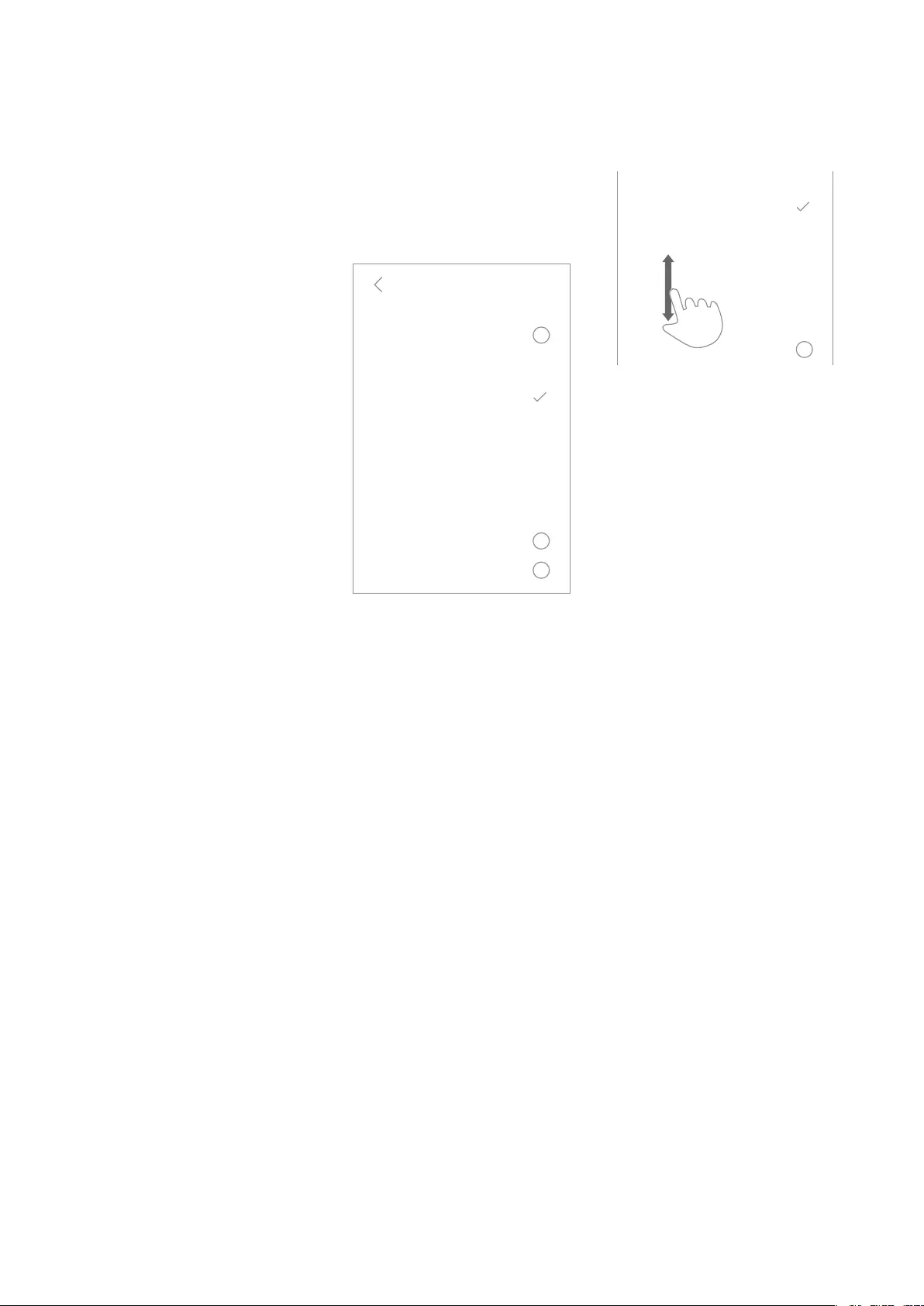
Inputs
As can be seen on the connector panel
shown in Figure 5.5, the BeoLab 50 has
a total of seven different audio inputs
as follows:
Bang & Olufsen Proprietary
•Power Link (analogue)
•Wireless Power Link (digital)
Digital inputs
•S/P-DIF (or “coaxial”)
•Optical
•USB Audio
Analogue inputs
•RCA Phono (or “unbalanced line”)
Wireless inputs
•WiSA
The technical specifications for these
can be found in Inputs.
It is possible to enable an audio source
connected to an input either manually
(via the BeoLab 50 interface) or
automatically, as described below.
5.1 Inputs Selection
5.1.1 Automatic Selection
Inputs
Power Link
S/P-DIF
RCA
Optical
USB-Audio
WPL / WiSA
Automatic
AUTOMATIC SENSE
MANUAL SENSE
... Inputs
Power Link
S/P-DIF
RCA
Optical
USB-Audio
WPL / WiSA
Automatic
AUTOMATIC SENSE
MANUAL SENSE
... RCA
Gain Offset 5 dB
-76 dBV
5 min.
50 kΩ
2.0 V
Detection threshold
Max input voltage
Input impedance
Time-out
Figure 5.1: The Input Select menu.
Selection Priority
If the BeoLab 50 is set to automatically
detect an input signal, then it may be
necessary to customise the
prioritisation of the sources. For
example, if you have a CD player
connected to the S/P-DIF input and a
turntable connected to the RCA input,
and both sources are playing, this
parameter allows you to determine
which source should “win” and be
played by the BeoLab 50.
This prioritisation can be personalised
by changing the vertical order of the
inputs on BeoLab 50 interface in the
Input Select menu (press the “...” icon
at the top right to enter the edit mode).
Inputs
Power Link
S/P-DIF
RCA
Optical
USB-Audio
WPL / WiSA
Automatic
AUTOMATIC SENSE
MANUAL SENSE
... Inputs
Power Link
S/P-DIF
RCA
Optical
USB-Audio
WPL / WiSA
Automatic
AUTOMATIC SENSE
MANUAL SENSE
... RCA
Gain Offset 5 dB
-76 dBV
5 min.
50 kΩ
2.0 V
Detection threshold
Max input voltage
Input impedance
Time-out
Figure 5.2: The priority of automatically-
selected sources can be changed by re-
arranging their order.
5.1.2 Manual Selection
There may be cases where you prefer
to manually select an input. In this
case, you can drag the input into the
“Manual Sense” list at the bottom of
the Inputs menu screen. In this case, a
signal on one of these inputs will not
be automatically detected by the
BeoLab 50 and must therefore be
switched on and off manually.
Note that it is possible to manually
select inputs without the interface
using the BeoRemote 1 remote control.
For a description of how to do this,
including instructions on setting up the
BeoRemote 1, please see Section 20.
5.2 Individual Input
Parameters
Note that not all controls are available
for all inputs.
5.2.1 Re-naming
It is possible to re-name the inputs
labels in the BeoLab 50 interface by
entering the edit mode of the Inputs
menu, selecting an input, and then
press-and-hold the name of the input
at the top of the screen.
This personalised name (i.e. “CD
Player” or “Turntable”, for example)
will be displayed throughout the
23
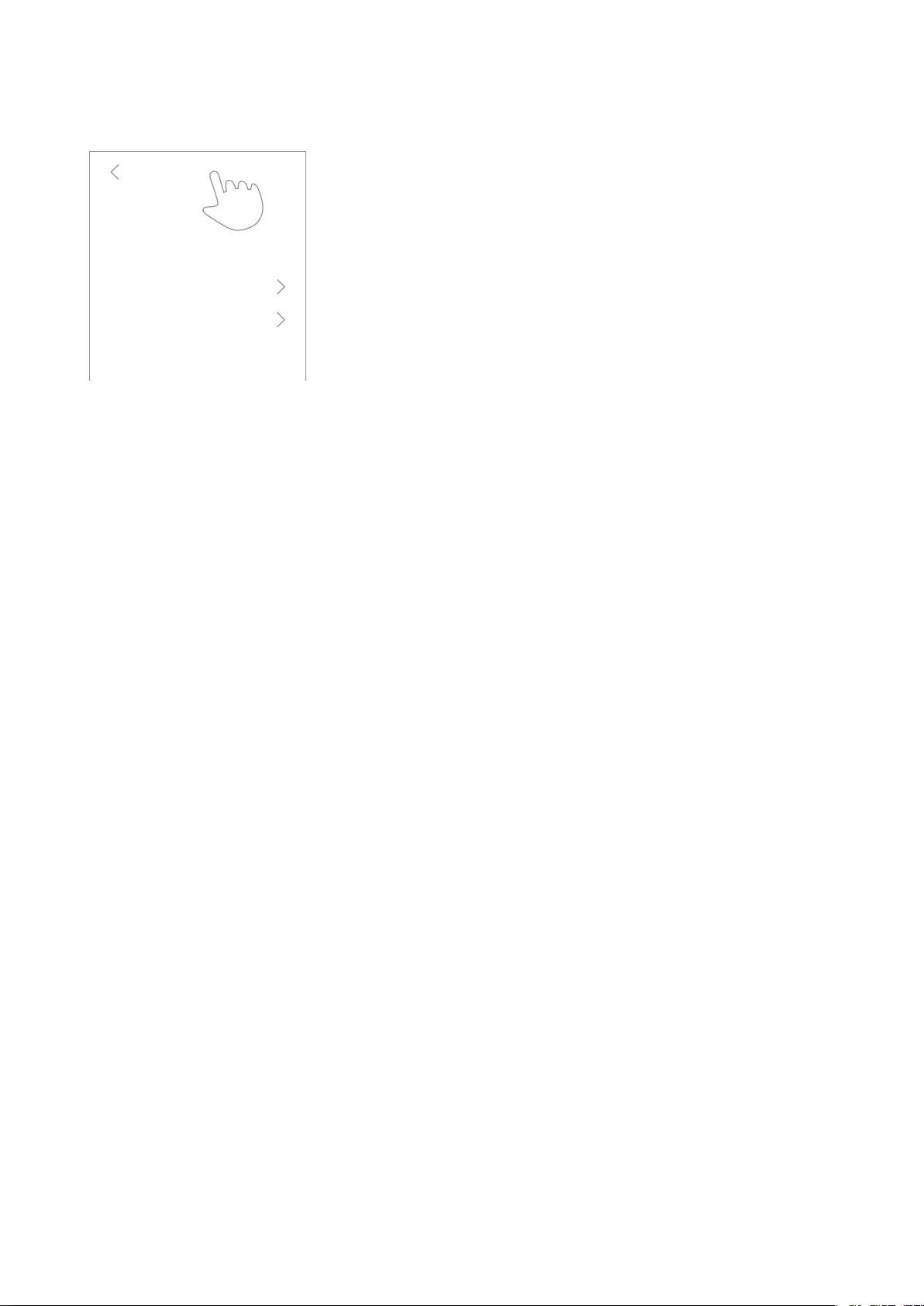
BeoLab 50 interface.
Inputs
Power Link
XLR
S/P-DIF
RCA
Optical
USB-Audio
WPL / WiSA
Automatic
AUTOMATIC SENSE
MANUAL SENSE
...
Inputs
Power Link
XLR
S/P-DIF
RCA
Optical
USB-Audio
WPL / WiSA
Automatic
AUTOMATIC SENSE
MANUAL SENSE
... RCA
Gain Offset 5 dB
-76 dBV
5 min.
50 kΩ
2.0 V
Detection threshold
Max input voltage
Input impedance
Time-out
Figure 5.3: Rename an input by pressing
and holding its name in the Input menu
5.2.2 Gain Offset
You can change the relative levels of
the individual inputs using the Gain
Offset parameter. For example, if you
have a particular source that has a
lower output level than the others, its
Gain Offset can be increase to
compensate, making it appear to have
the same level as your other audio
sources.
Range -12 to 12 dB
Resolution 1 dB
Factory Default 0 dB
Note that the Gain Offset parameter is
not available for the Power Link and
Wireless Power Link inputs.
5.2.3 Detection Threshold
The BeoLab 50 can be set to
automatically turn itself on by
detecting the presence of a signal on
the RCA line input. However,
depending on your source and/or the
style of music you typically listen to, it
may be necessary to make the
detection more or less sensitive. This
can be done using the Detection
Threshold control.
For example, if you listen to music with
a large dynamic range, it may be
necessary to lower the Detection
Threshold to make the BeoLab 50 more
sensitive to the presence of quiet
signals. Conversely, if you have an
audio source that has a higher noise
floor, it may be necessary to increase
the Detection Threshold in order to
make the BeoLab 50 less sensitive.
Range -76 to -46 dBV
Resolution 3 dB
Factory Default -76 dBV
See Figure 5.4 for a graphic
representation of the the detection
threshold relative to the signal
strength.
Note that the Detection Threshold
parameter is not available for the
Power Link input, since the
loudspeaker is automatically turned on
and off by the Power Link source.
Note that, for the USB Audio, S/P-DIF
and Optical digital inputs, the
Auto-detection control is used instead
of the Detection Threshold.
5.2.4 Auto-detection
The BeoLab 50 can be set to
automatically turn itself on by
detecting the presence of a signal on
its digital inputs by setting the
Auto-detection to ON. However, this is
slightly different from the detection of
analogue signals, since the digital
inputs are triggered by the presence of
a any non-zero signal on the digital
audio stream rather than a signal
above a user-defined level .
Options On / Off
Factory Default On
Note that the Auto-detection
parameter is only available for the USB
Audio, S/P-DIF and Optical inputs.
Note that, for the RCA analogue input,
the Detection Threshold control is used
instead of the Auto-detection.
5.2.5 Maximum Input Voltage
Different audio sources have different
maximum analogue output levels.
Typically, a maximum level from a
line-level RCA output is 2.0 V RMS,
however, different manufacturers
occasionally choose to deliver a higher
output level on some models.
In order to maximise the
signal-to-noise ratio of your audio
system, the BeoLab 50 gives you the
option to change the Maximum Input
Voltage for the the RCA line input. The
datasheet for your audio source should
indicate its maximum output level. The
value in the BeoLab 50 interface
should be set to match this value.
If the source has a higher maximum
output level than that which is set in
the BeoLab 50 interface, this may
cause distortion due to clipping of the
signal at the loudspeaker’s inputs.
If the source has a lower maximum
output level than that which is set in
the BeoLab 50 interface, this will cause
your maximum output of the
loudspeaker to be lower, and the
output noise floor to be increased.
Options 2.0, 4.0, 6.5 V RMS
Factory Default 2.0 V RMS
Note that the Maximum Input Voltage
parameter is only available for the RCA
line input.
5.2.6 Time-out
In cases where the automatic signal
detection is used to turn the on BeoLab
50, the Time Out control can be used
to determine the length of time the
loudspeaker continues to be powered
up after the audio signal has stopped.
It may be necessary to increase the
length of this time if you listen to
music with an extreme dynamic range.
For example, a quiet passage in a
piece of music may be below the
detection threshold. If the duration of
that passage is longer than the Time
Out value, then the loudspeaker will go
into standby mode while the piece is
playing.
Options 2, 5, 10, 15, 20 Minutes
Factory Default 15 Minutes
Note that the Time-out function is not
available for the Power Link, Wireless
Power Link and WiSA inputs.
24
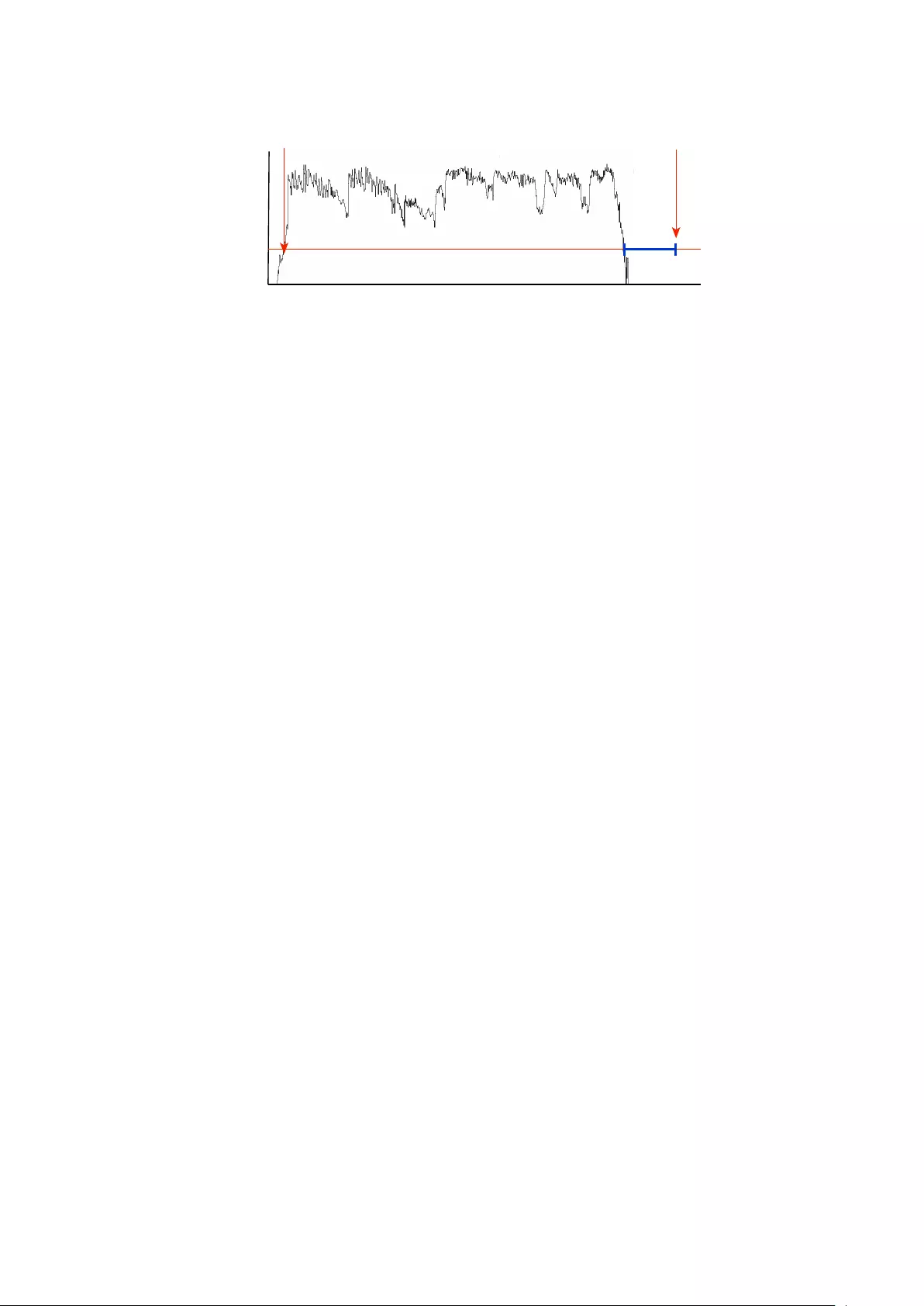
Detection
Threshold
BeoLab 90
turns ON
BeoLab 90
turns OFF
Time Out
Audio level at input
Time
Audio Signal
Figure 5.4: The “Detection Threshold” and “Time Out” parameters
5.2.7 Input Impedance
If the BeoLab 50’s RCA Line input is
connected to a device’s headphone
output that uses a Class-D amplifier,
there may be instances where this
causes the noise floor to rise audibly.
This is caused by the input impedance
of the BeoLab 50 being much higher
than that which is expected by the
headphone amplifier’s designer. In
order to correct this problem, the input
impedance of the RCA input can be set
to a low value of 50 Ω.
However, if the input impedance of the
RCA input is set to 50 Ωand it is
connected to a device’s standard
low-impedance line output, this may
have a detrimental effect on the signal.
For example, the maximum possible
output level will be reduced. In some
cases, incorrectly setting the input
impedance to 50 Ωmay also cause
distortion of the audio signal.
Options 50 Ω, 50 kΩ
Factory Default 50 kΩ
Note that the Input Impedance control
is only available for the RCA line input.
5.2.8 Control Volume of
S/P-DIF (or Optical)
input using Power Link
Bang & Olufsen audio products that are
able to send the audio signal on an
S/P-DIF output additionally send the
volume setting on the data connection
included in the Power Link cable. This is
used for various reasons. One primary
example of this is current customers
who connect a BeoSound 9000 to a
pair of loudspeakers such as BeoLab
5’s or BeoLab 50’s via a S/P-DIF digital
connection. Since the BeoSound 9000
does not apply volume regulation to
the S/P-DIF output, the volume setting
must be sent separately on the Power
Link cable and applied to the audio
signal inside the loudspeaker instead.
This parameter on the BeoLab 50
allows customers to use the volume
control of a Bang & Olufsen source
(sent via a Power Link connection) and
apply it to an audio signal coming into
the BeoLab 50 via its S/P-DIF input.
Note that, in order for this option to
function properly, the S/P-DIF input
must be assigned a higher priority than
the Power Link input in the Selection
Priority.
This function is also independently
available for signals on the Optical
input.
When the volume of the BeoLab 50 is
controlled by an external Power Link
source, the volume wheel in the
BeoLab 50 interface is greyed out and
will not respond to touch commands. It
does, however, display the volume
setting assigned to the BeoLab 50 by
the Power Link data signal.
Options Enabled / Disabled
Factory Default Disabled
5.2.9 USB Volume enabled
When the BeoLab 50 is connected
using USB Audio to an audio source,
you have the option of using the
source’s volume as an external control
for the gain of the loudspeaker. This
also means that the volume of the
BeoLab 50 (set by its remote control)
would be reflected on the user
interface of the audio source or
software player.
However, this external control of the
BeoLab 50 may not be desirable in all
situations. For example, it is very easy
to instantly change the volume of a
software audio player to maximum,
which will be surprisingly loud with a
BeoLab 50 if the change was
accidental. It also may be preferable to
set the BeoLab 50 to a static (e.g. low)
volume setting and to have an
independent adjustment on the source
device. In these cases, the USB Volume
Enabled should be set to Disable.
Options Enabled / Disabled
Factory Default Disabled
Note that the USB Volume control is
only available for the USB Audio input.
5.3 Connection Panels
The connection panels on the Master
and Slave BeoLab 50’s are slightly
different in that audio signals can only
be connected to the Master
loudspeaker. The audio signal
connections from your source devices
should be connected to the Master
loudspeaker. The only audio input on
the Slave loudspeaker is the DPL or
Digital Power Link input.
25
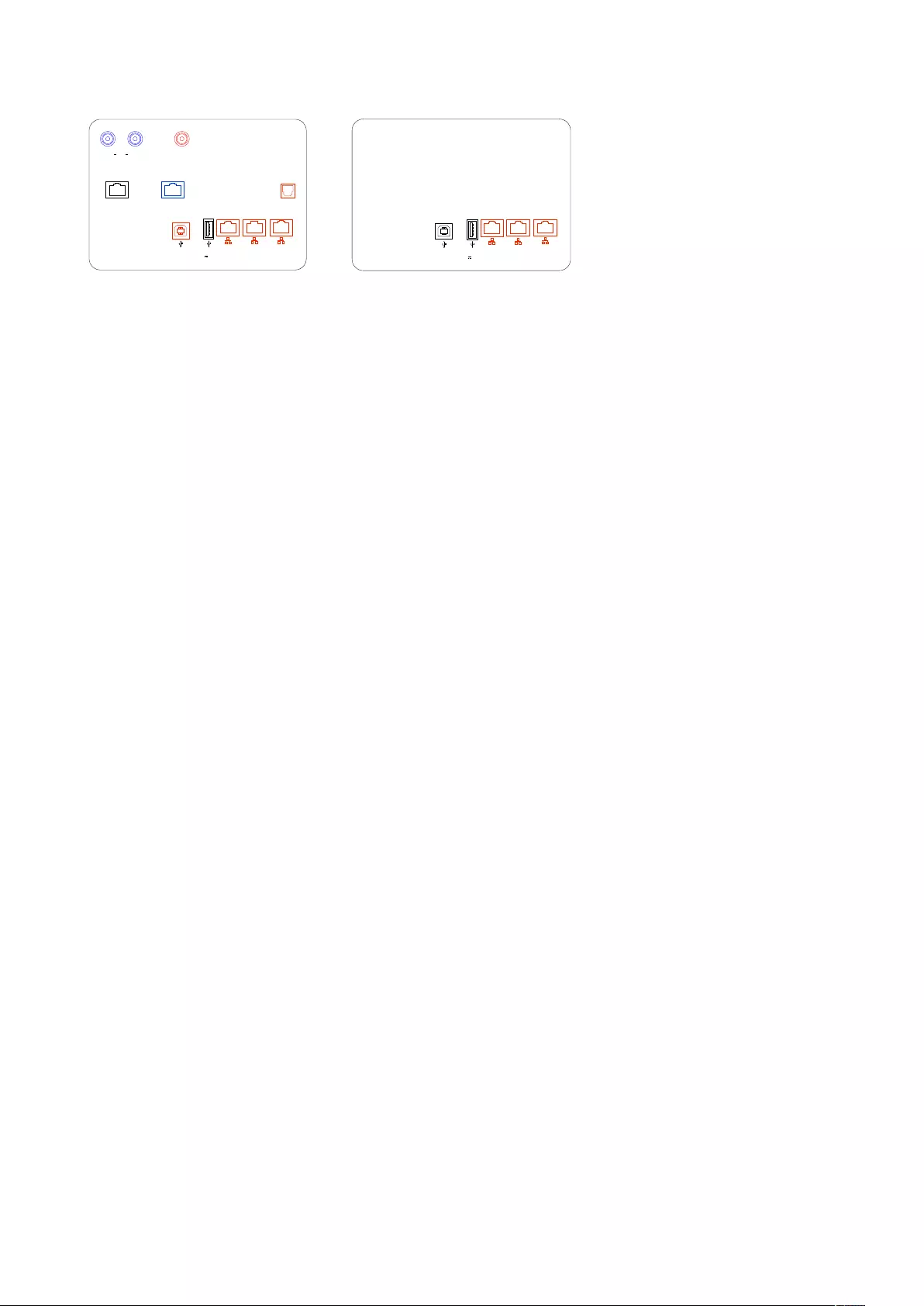
OPTICAL
S/P-DIF
MIC / IR POWER LINK
LEFT RCA RIGHT
USB
5V 0.5 A
USB
AUDIO
DPL DPL DPL /
ETHERNET
USB
5V 0.5 A
DPL /
ETHERNET
DPL DPL
Figure 5.5: Audio connection panel –
Master loudspeaker. Analogue inputs
are shown in blue. Digital audio con-
nections are shown in red. Utility con-
nections are shown in black.
OPTICAL
S/P-DIF
LEFT XLR RIGHT
MIC / IR POWER LINK
LEFT RCA RIGHT
USB
5V 0.5 A
USB
AUDIO
DPL DPL DPL /
ETHERNET
USB
5V 0.5 A
DPL /
ETHERNET
DPL DPL
Figure 5.6: Audio connection panel –
Slave loudspeaker. Note that the only
audio connectors on this loudspeaker
are for the Digital Power Link connection
to the Master loudspeaker.
For specific information regarding the
various inputs, please see Inputs.
26
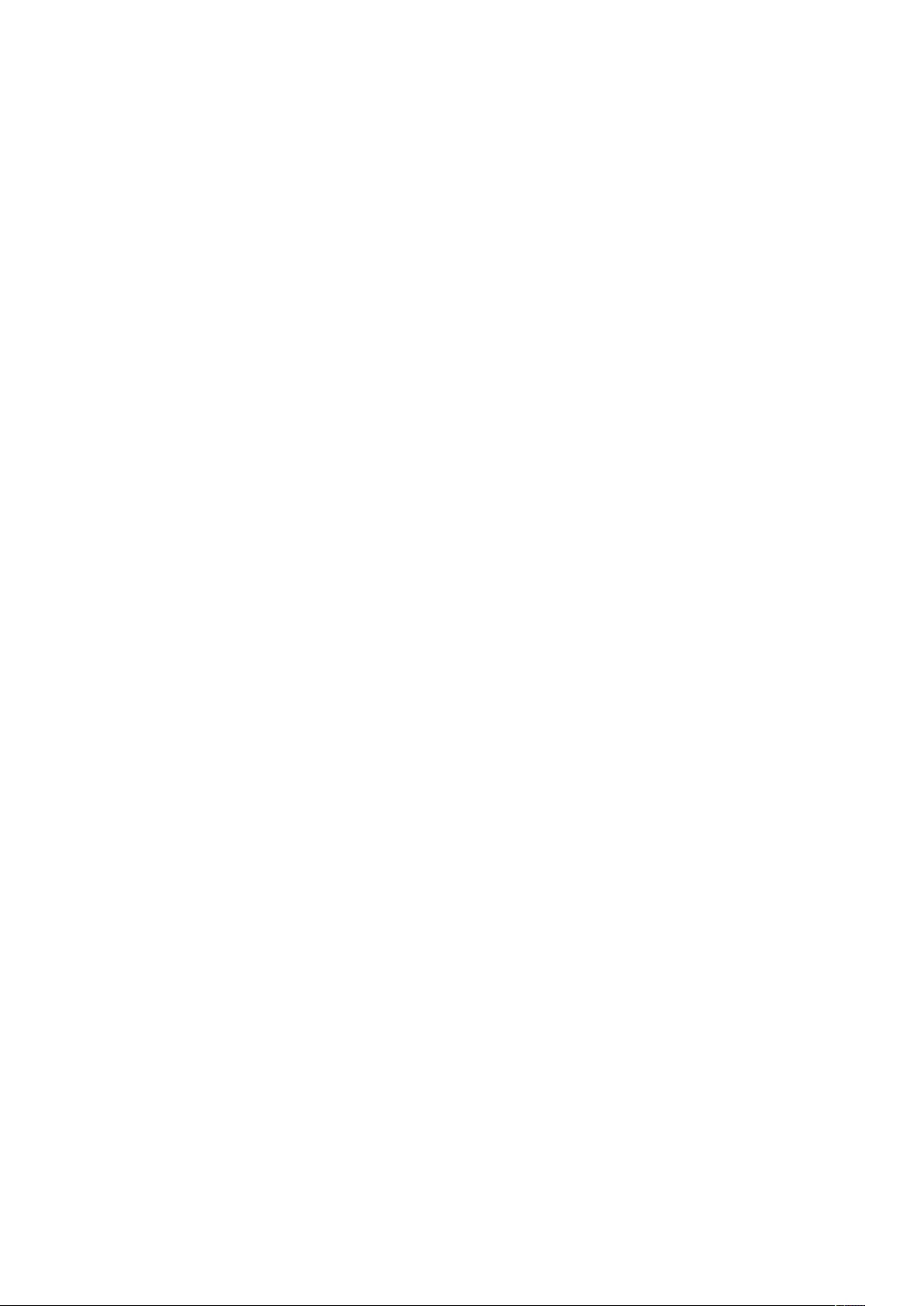
System
6.0.1 About
The “About” menu allows access to
information regarding the loudspeaker
as well as the audio signal it is
currently playing. Select the “Speaker
Info”, and then either the “Input
Signal” to display detailed information
about the input audio signal or
“Temperatures” to display the current
temperatures of the loudspeaker
drivers.
6.0.2 Max Volume
The Max Volume control allows you to
determine the limit of the volume
control.
Range 0 – 90
Resolution 1 dB
Factory Default 90
Note that the Max Volume parameter is
not available for the Power Link and
Wireless Power Link inputs.
6.0.3 Startup Volume
The Startup Volume control allows you
to determine the volume level when
the BeoLab 50 wakes as a result of a
detected signal, or is manually turned
on.
Range 0 – 90
Resolution 1 dB
Factory Default 42
Note that the Startup Volume
parameter is not available for the
Power Link and Wireless Power Link
inputs. Also note that the Startup
Volume may be overridden by the
volume control from Power Link (if
enabled for S/P-DIF or Optical) or a USB
Audio volume control (if enabled).
27
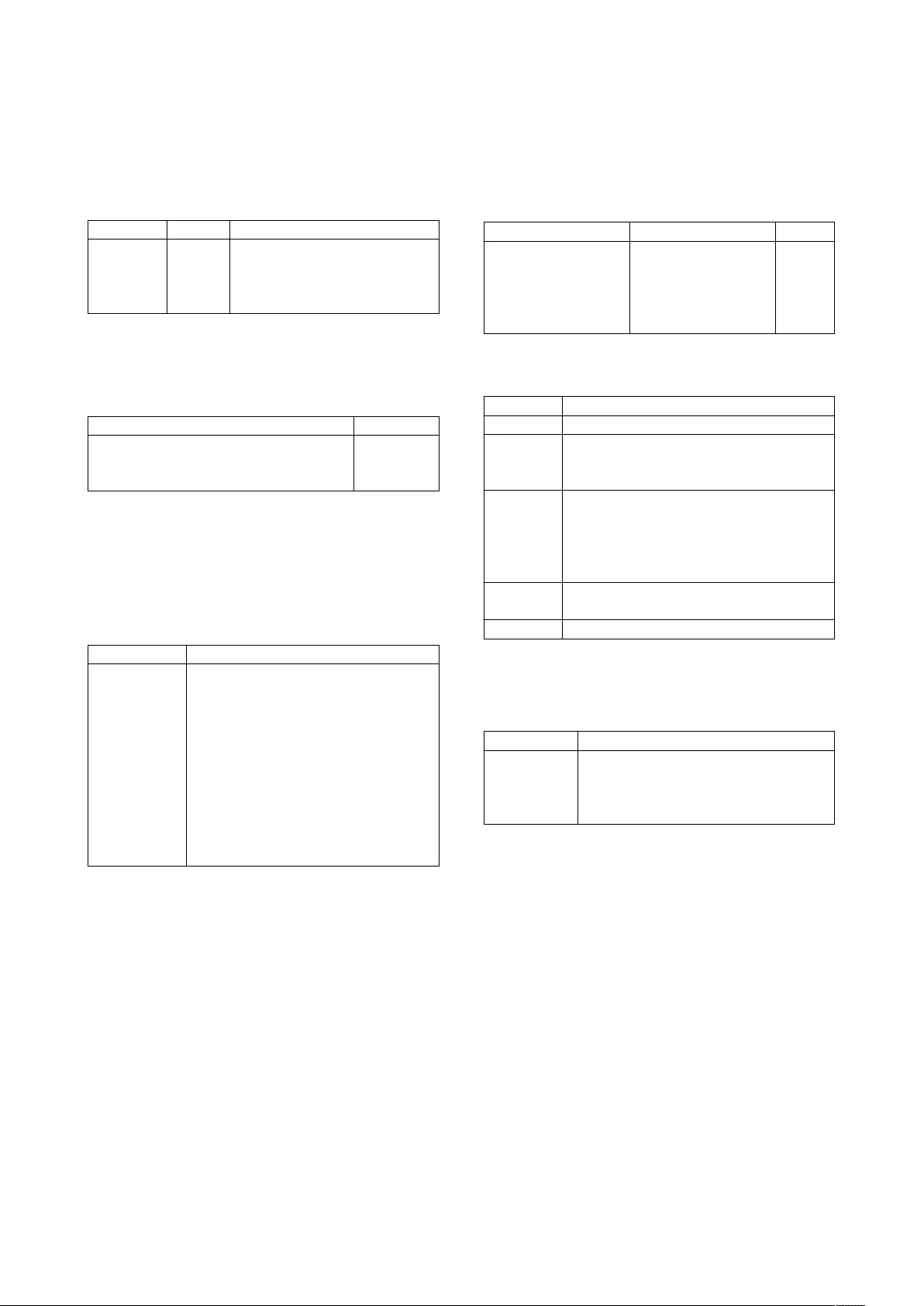
Tables
7.1 Loudspeaker Sensitivity
Input dB SPL “Max Input” Level Setting
Power Link 88.0 6.5 V rms (Fixed)
RCA 88.0 6.5 V rms
RCA 92.2 4.0 V rms
RCA 98.2 2.0 V rms
Table 7.1: Unweighted Sound Pressure Level (SPL) of the Be-
oLab 50 at 1 m in a free field (200 Hz – 2 kHz). Input signal
strength: 125 mV rms. Volume Step: 90. All other parameters
set to Factory Defaults.
Input Output
USB Audio, S/P-DIF, Optical 92.3 dB SPL
Wireless Power Link 92.3 dB SPL
WiSA TBD
Table 7.2: Unweighted Sound Pressure Level (SPL) of the Beo-
Lab 50 at 1 m in a free field (200 Hz – 2 kHz). Input signal: -30.0
dB FS. Volume Step: 90. All other parameters set to Factory
Defaults.
7.2 Volume Control
Volume Step Output
90 92.3 dB SPL
89 91.3 dB SPL
88 90.3 dB SPL
. .
51 53.3 dB SPL
50 52.3 dB SPL
49 51.3 dB SPL
. .
2 4.3 dB SPL
1 3.3 dB SPL
0 -∞dB SPL
Table 7.3: Unweighted Sound Pressure Level (SPL) of the audio
signal from a BeoLab 50 at 1 m in a free field (200 Hz – 2 kHz).
Input signal: -30.0 dB FS. Note that these values consider only
the output level of the audio signal and assume that thermal
protection has not been engaged.
7.3 Parametric Equaliser
Type Range (Hz) Filters
low-shelving 16.0 – 500.0 1
Peaking (LF) 16.0 – 500.0 4
Peaking (MF) 250.0 – 8.0 3
Peaking (HF) 2.0 k – 63.0 k 1
high-shelving 500.0 – 16.0 k 1
Table 7.4: Frequency ranges of Parametric EQ filters.
Band Frequency (Hz)
Ultrasonic 31.5k 35.5k 40k 45k 50k 56k 63k
Treble 16k 18k 20k 22.4k 25k 28k
8k 9k 10k 11.2k 12.5k 14k
4k 4.5k 5k 5.6k 6.3k 7.1k
Midrange 2k 2.24k 2.5k 2.8k 3.15k 3.55k
1k 1.12k 1.25k 1.4k 1.6k 1.8k
500 560 630 710 800 900
250 280 315 355 400 450
125 140 160 180 200 224
Bass 63.0 71.0 80.0 90.0 100 112
31.5 35.5 40.0 45.0 50.0 56.0
Infrasound 16.0 18.0 20.0 22.4 25.0 28.0
Table 7.5: ISO 1/6th octave-spaced centre frequencies (in Hz)
of Parametric EQ filters. Frequency Bands are given for approx-
imate information only.
Filter Type Q values
low-shelving 0.35, 0.5, 0.7, 1.0
Peaking 0.35, 0.5, 0.7, 1.0, 1.4,
2.0, 2.8, 4.0, 5.6, 8.0
high-shelving 0.35, 0.5, 0.7, 1.0
Table 7.6: Available Q values of Parametric EQ filters.
28
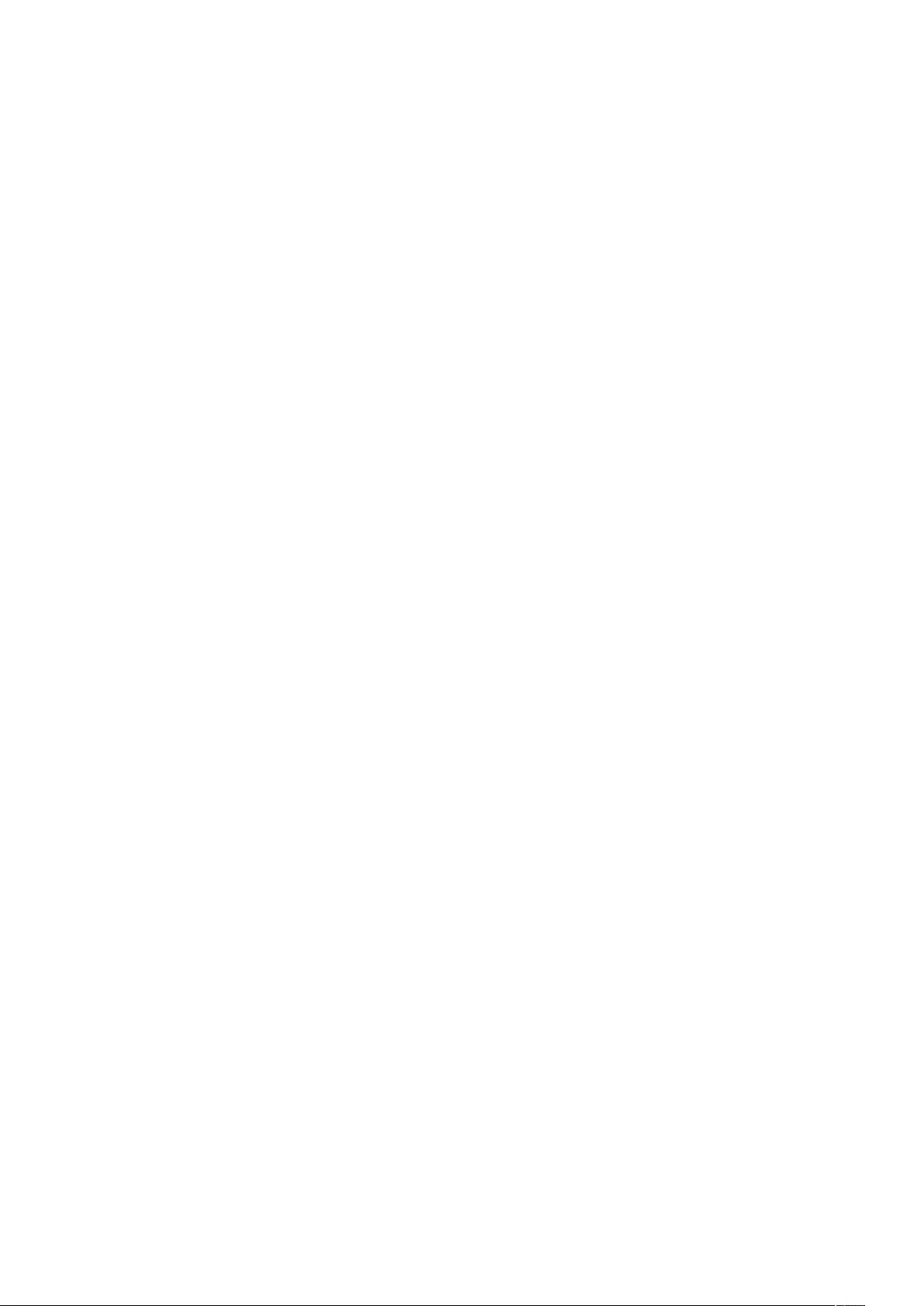
Features
8.1 Resonance-based Sound
Design
A very large part of the sound tuning of
the BeoLab 50, like many other Bang &
Olufsen loudspeakers, is based on
acoustical measurements performed at
many locations around, above and
below the loudspeaker.
One of the important aspects of these
measurements is to find the behaviour
of the loudspeaker in time. For
example, if a sound is sent to the
loudspeaker, and then stopped
suddenly, does the loudspeaker also
stop, or does it “ring” at some
frequencies (in exactly the same way
that a bell rings when struck). Ringing
in the time response of a loudspeaker
is an indication that it has a resonance
– a frequency at which it “wants” to
move. This resonance has a
detrimental effect on the overall sound
of the loudspeaker, since it “smears”
sounds in time.
For example, if you have a loudspeaker
that has a natural resonance at 110 Hz
(two octaves below a “Concert A”, to
musicians) then it will naturally ring at
that note when it is “hit” with an
impulsive signal such as a kick drum. If
the song that the band is playing is not
in A (major or minor), but in B-Flat
instead, then there will be a
dissonance between the notes played
most often in the song, and the note
that is “singing along” with the kick
drum. This can contribute to the
loudspeaker sounding “muddy” (to use
only one word...).
This is why the measurement-based
portion of the filtering of all current
Bang & Olufsen loudspeakers is
primarily designed to counteract the
natural resonances in the system. So,
for example, if one of the woofers in
the BeoLab 50 has a natural resonance
at 110 Hz, then that resonance is
mirrored with an equal, but opposite
phase behaviour in the Digital Signal
Processing engine. The total result of
the filter in the DSP and the behaviour
of the woofer is that there is no
unwanted ringing in the entire system.
This, in turn, means that the
loudspeaker’s response is controlled
not only in the frequency domain but in
the time domain as well.
This is only possible with an extensive
set of measurements of each
loudspeaker driver’s mechanical and
acoustical behaviour and a
custom-created set of filters for it.
8.2 Phase-Optimised Filtering
Like all audio devices, in order for the
BeoLab 50 to deliver its level of sound
performance, filters are used in the
Digital Signal Processing (DSP).
Generally, an audio filter is a device
that changes the overall response of
the the audio signal. In the case of
BeoLab 50, these are used for various
reasons such as controlling the
relationship between the different
loudspeaker drivers, acting as
crossovers to distribute the correct
signals to the tweeters, midranges and
woofers, and optimising the overall
magnitude response of the total
system.
An audio filter has an effect on the
behaviour of the signal’s magnitude
(how loud it is at a given frequency)
and/or its phase (a type of measure of
the amount of time it takes a given
frequency to get through the filter).
Since the BeoLab 50 uses digital
instead of analogue filters, we are able
to choose the characteristics of each
filter’s phase response independently
of its magnitude response. For
example, a filter can be implemented
to have a “minimum phase” or a
“linear phase” (the two most common
responses) characteristic, regardless of
the magnitude response it is required
to deliver.
The phase response of each filter in
BeoLab 50’s processing chain have
been individually tailored according to
its particular function. For example,
some of the crossover filters have been
implemented as linear phase filters.
Most filters in the Active Room
Compensation algorithm are
implemented as minimum phase filters
(since room resonances have a
minimum phase characteristic). The
Beam Width Control filters have
customised phase responses that are
dependent on the particular
frequency-dependendent
characteristics of the individual
loudspeaker drivers that they control
and are therefore neither minimum
phase nor linear phase.
8.3 Automatic Bass
Linearisation (ABL) and
Thermal Protection
Almost all loudspeakers in the Bang &
Olufsen portfolio (including BeoLab 50)
feature Automatic Bass Linearisation or
“ABL”. This is an algorithm that was
patented by B&O in 1991 and is
custom-tuned for each of our products.
Its purpose is to ensure that, when the
physical limits of a component of the
loudspeaker are reached (for example,
a woofer is approaching its maximum
excursion, or a power amplifier is close
to clipping) the loudspeaker either
prevents that limit from being reached,
or the transition to that limit is
“softened” (depending on the
component in question).
In addition, BeoLab 50’s processing
continually monitors the individual
temperatures of many internal
components including:
•Individual loudspeaker driver
magnets
•Power Amplifier modules
•DSP circuit boards
•Power Supply circuit boards
Using this information, combined with
the power that the amplifiers deliver to
the loudspeaker drivers, the
29
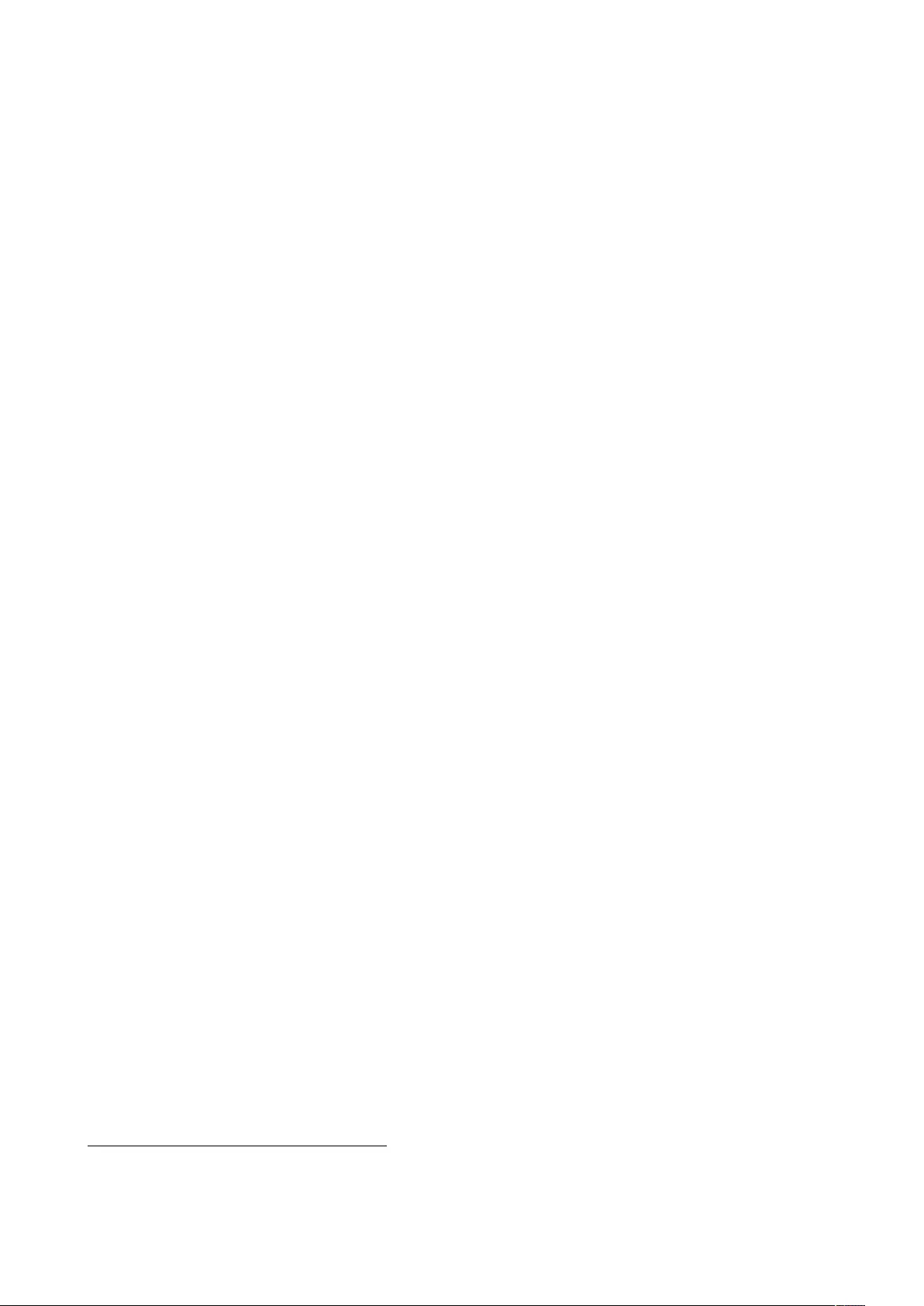
temperatures of many more
components within BeoLab 50 are
calculated using customised thermal
models of the loudspeaker.
If the temperature of a component
inside the loudspeaker approaches its
“thermal limit” (the temperature at
which it stops working due to
overheating) the signal processing of
the BeoLab 50 adjusts the signals to
protect the component. The exact type
of adjustment depends on the
particular component that is
approaching its limits. As a simple
example, if a tweeter voice coil is
calculated to be approaching its limit,
then its gain is reduced to attempt to
protect it from destruction.
It is important to state that this does
not mean that the BeoLab 50 is
indestructible – but it does make it
very difficult to destroy.
More information can be found in
Appendix 6: ABL - Adaptive Bass
Linearisation.
8.4 Thermal Compression
Compensation
BeoLab 50’s processing includes
automatic compensation for changes in
loudspeaker driver response as a result
of internal changes in temperature.
For more an in-depth discussion of this
feature, please read Appendix 7:
Thermal Compression Compensation.
8.5 Production “Cloning”
Every BeoLab 50 that leaves the
production line is measured in a
custom-built anechoic chamber to
ensure that its performance matches
the master reference loudspeaker. This
automated measurement is performed
using a number of microphones where
small differences in the responses are
found and custom correction filters are
created and loaded into the Digital
Signal Processing. This ensures that
each loudspeaker’s third-octave
smoothed response matches that of
the master reference loudspeaker
within 1 dB between 20 Hz and 20
kHz.1
1Note that these values have not yet been finalised.
30
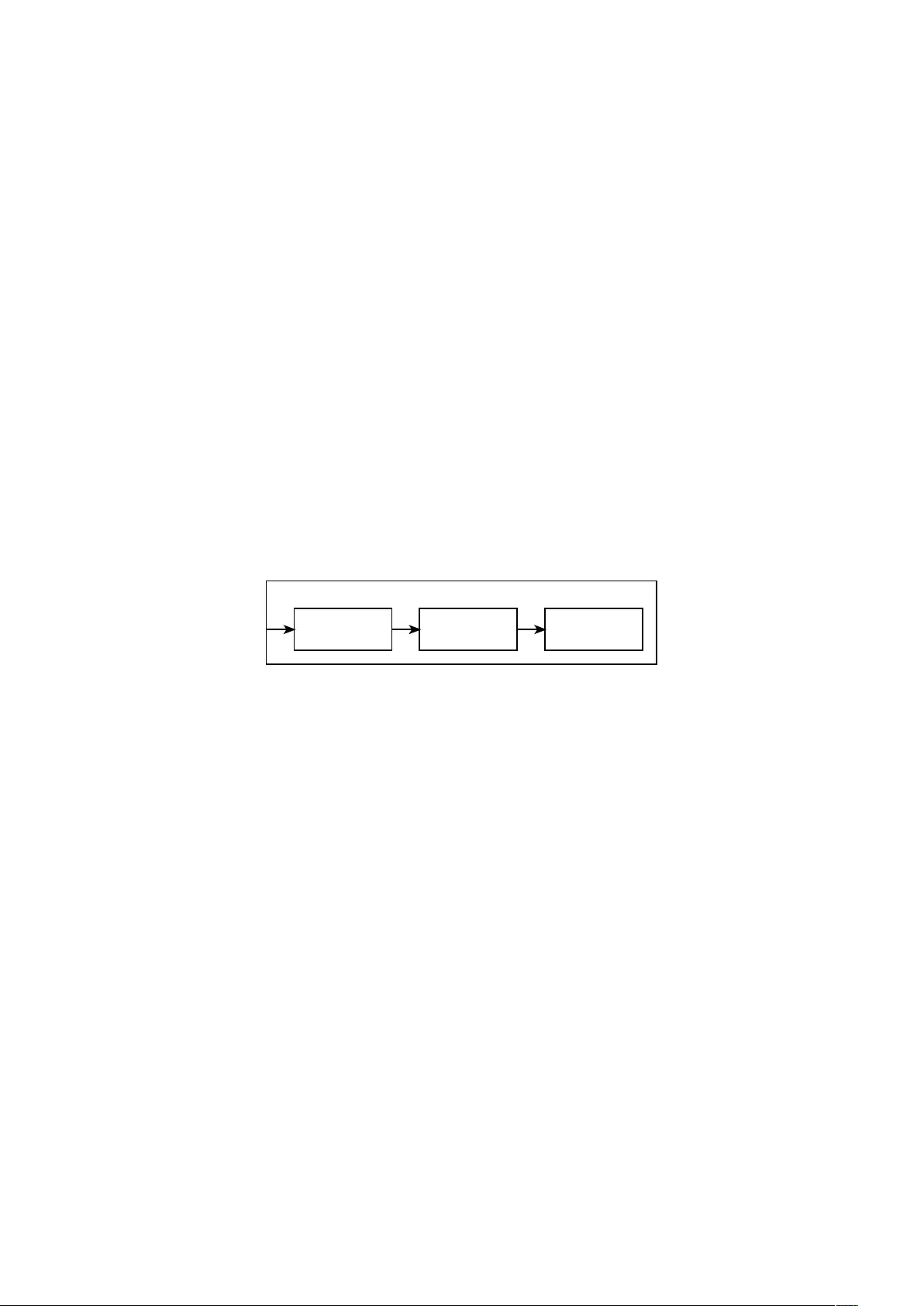
Technical Specifications
9.1 Total System
Note: Total System measurements performed with Sound Design set to “Flat on-axis” and Active Room Compensation disabled.
Frequency Response XX Hz to XX kHz (±1 dB, 1/3 octave smoothed)
Frequency Range XX Hz to XX kHz (-10 dB, ref. 200 Hz - 2 kHz, unsmoothed)
Sensitivity see Section 7.1
Maximum SPL XX dB SPL (C) @ 1 m, on-axis
Self Noise (Digital input) XX dB SPL (C) @ 1 m, on-axis
Self Noise (Analogue input) XX dB SPL (C) @ 1 m, on-axis
9.2 Preamplifier and Processor Section
In order to simplify comparison of BeoLab 50’s technical data to other products, the information in this chapter has been divided into
three sections:
•Preamplifier and Processor, equivalent to a surround processor, preamp or receiver
•Power Amplifiers
•Loudspeaker Drivers
Stereo Preamp
BeoLab 90
Power Amps Loudspeakers
Figure 9.1: A block diagram of the BeoLab 50 showing the comparative sections in terms of competing devices.
9.2.1 Overall Specifications
Note: Hardware-only measurement. All filters and equalisation bypassed or removed from signal processing for measurements.
Digital input to DAC outputs
Frequency Response 0 Hz to 40 kHz (+ 0 dB, -1 dB)
Frequency Range 0 Hz to 75 kHz (+ 0 dB, -3 dB)
THD+N 0.004% (997 Hz, -1 dB FS, 22 Hz – 20 kHz)
Dynamic Range 122 dB (A) (997 Hz, -60 dB FS, 20Hz – 20kHz, AES17)
Analogue input to DAC outputs
Frequency Response TBD (+ 0 dB, -1 dB)
Frequency Range TBD (+ 0 dB, -3 dB)
THD+N TBD (997 Hz, -1 dB FS, 22 Hz – 20 kHz)
Dynamic Range TBD (A) (997 Hz, -60 dB FS, 20Hz – 20kHz, AES17)
31
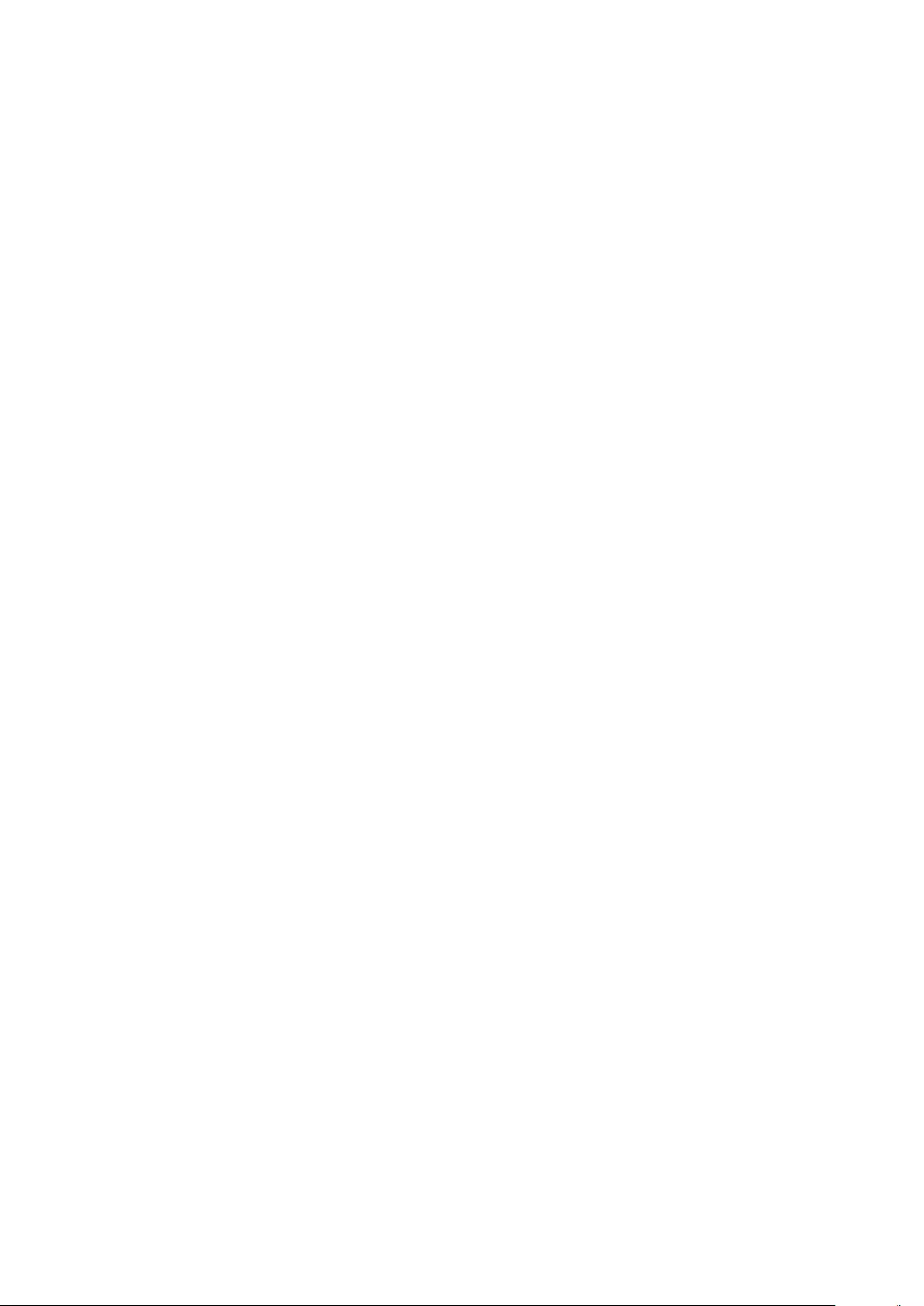
9.2.2 Inputs
Analogue Inputs
Analogue-to-Digital Converter
Note that the same ADC model is used for all analogue inputs.
Model Texas Instruments PCM4220
Sampling Rate 192 kHz (fixed)
Resolution 24 bits
Frequency Response 10 Hz – 80 kHz (+ 0 dB, -0.2 dB)
Frequency Range <2 Hz – 85 kHz (-3 dB)
Dynamic Range (Typical) 122 dB (A) (997 Hz, -60 dB FS)
Dynamic Range (Worst-case) 117 dB (A) (997 Hz, -60 dB FS)
THD+N 0.001% (997 Hz, -1 dB FS, 22 Hz – 20 kHz)
Channel Separation 100 dB (20 Hz to 20 kHz)
Passband Ripple ±0.001 dB
Power Link
Connector RJ45
Input Impedance 100 kΩ
Audio Channels 2
Maximum Input Voltage 6.5 V RMS
Features 5 V control voltage for On/Standby
Power Link Data support
Sensitivity 125 mV RMS produces 88 dB SPL (1 m, on-axis, free-field)
RCA Line
Input Impedance 50 Ω, 50 kΩ(Selectable)
Maximum Input Voltage 2.0, 4.0, 6.5 V RMS (Selectable)
Digital Inputs
Sampling Rate Converter
Note that the SRC is applied to all digital inputs.
Model Texas Instruments SRC4392
Output sampling rate 192 kHz (fixed)
Output word length 24 bits
THD+N 0.000014% (f=997 Hz, 0 dBFS, 22 Hz – 40 kHz, unweighted)
Dynamic Range 138 dB (f=997 Hz, -60 dBFS, 22 Hz – 40 kHz, unweighted)
Passband Ripple ±0.008 dB
32
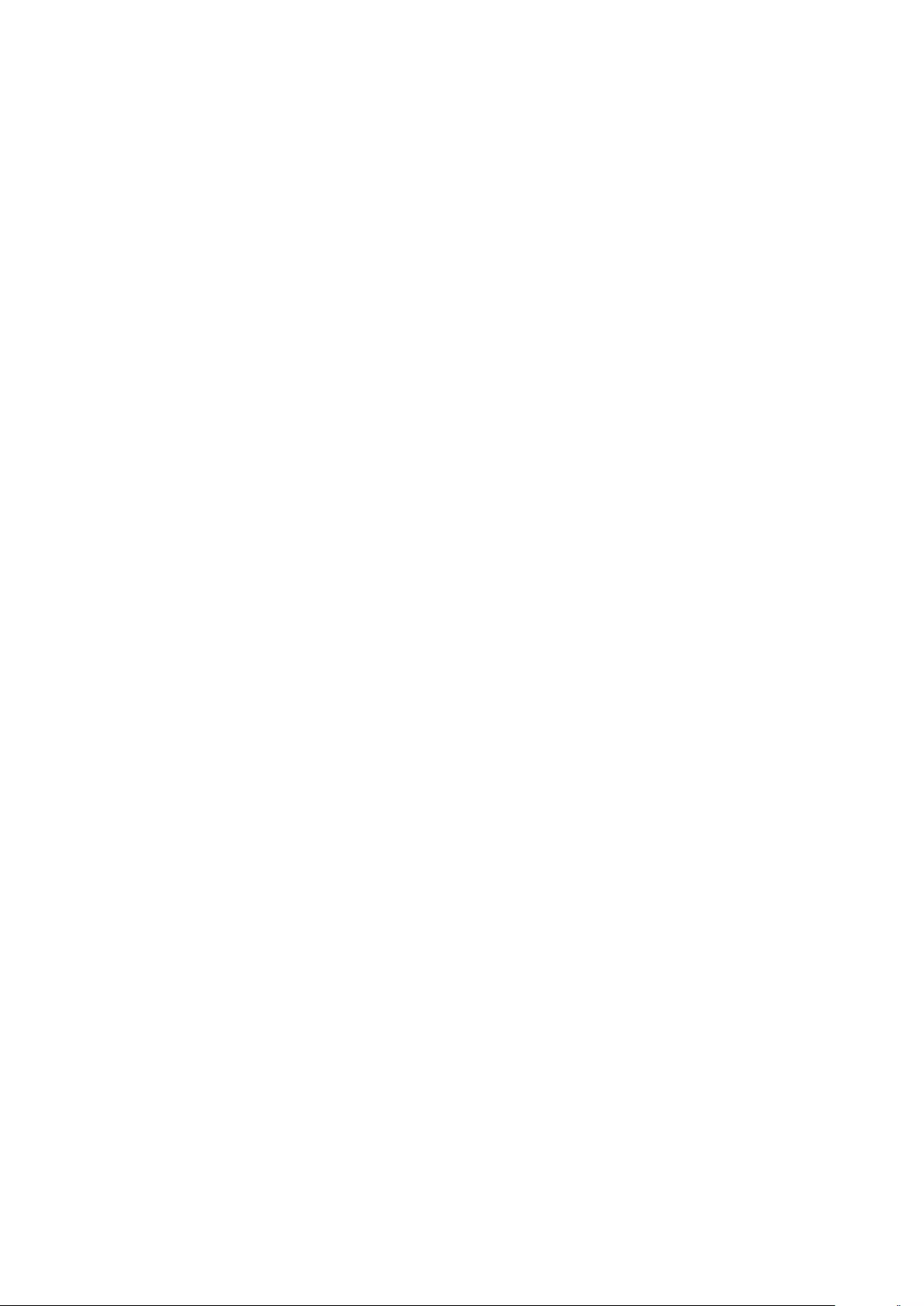
S/P-DIF
Supported Format Linear PCM
Sampling rate Standard sampling rates up to 192 kHz
Word length 24 bits
Optical Connector
Supported Format Linear PCM
Sampling rate Standard sampling rates up to and including 96 kHz
Word length 24 bits
USB Audio Connector
Supported Format Linear PCM
Sampling rate Standard sampling rates up to and including 192 kHz
Word length 24 bits
Wireless Power Link
Supported Format Linear PCM
Sampling rate Standard WiSA sampling rates up to and including 96 kHz (48 kHz standard)
Word length 24 bits
WiSA
Supported Format Linear PCM
Sampling rate Standard WiSA sampling rates up to and including 96 kHz
Word length 24 bits
9.2.3 Digital Signal Processor
Model Analog Devices ADSP-21489
Number 2
Instruction Rate 400 MHz
Sampling rate 192 kHz (fixed)
Notes 32-bit floating point
9.2.4 Digital to Analogue Converters
Note that these specifications include the analogue stages that follow the DAC outputs.
Model Texas Instruments / Burr-Brown PCM1798
Audio Channels 18
Sampling rate 192 kHz (fixed)
Word length 24 bits
Frequency Response 0 Hz to 40 kHz (+ 0 dB, -1 dB)
Frequency Range 0 Hz to 75 kHz (+ 0 dB, -3 dB)
THD+N 0.004% (997 Hz, -1 dB FS, 22 Hz – 20 kHz)
Dynamic Range 122 dB (A) (997 Hz, -60 dB FS, 20Hz – 20kHz, AES17)
Channel Separation 110 dB (20Hz – 20kHz, AES17)
Level Linearity ±1 dB (at -120 dB FS)
33
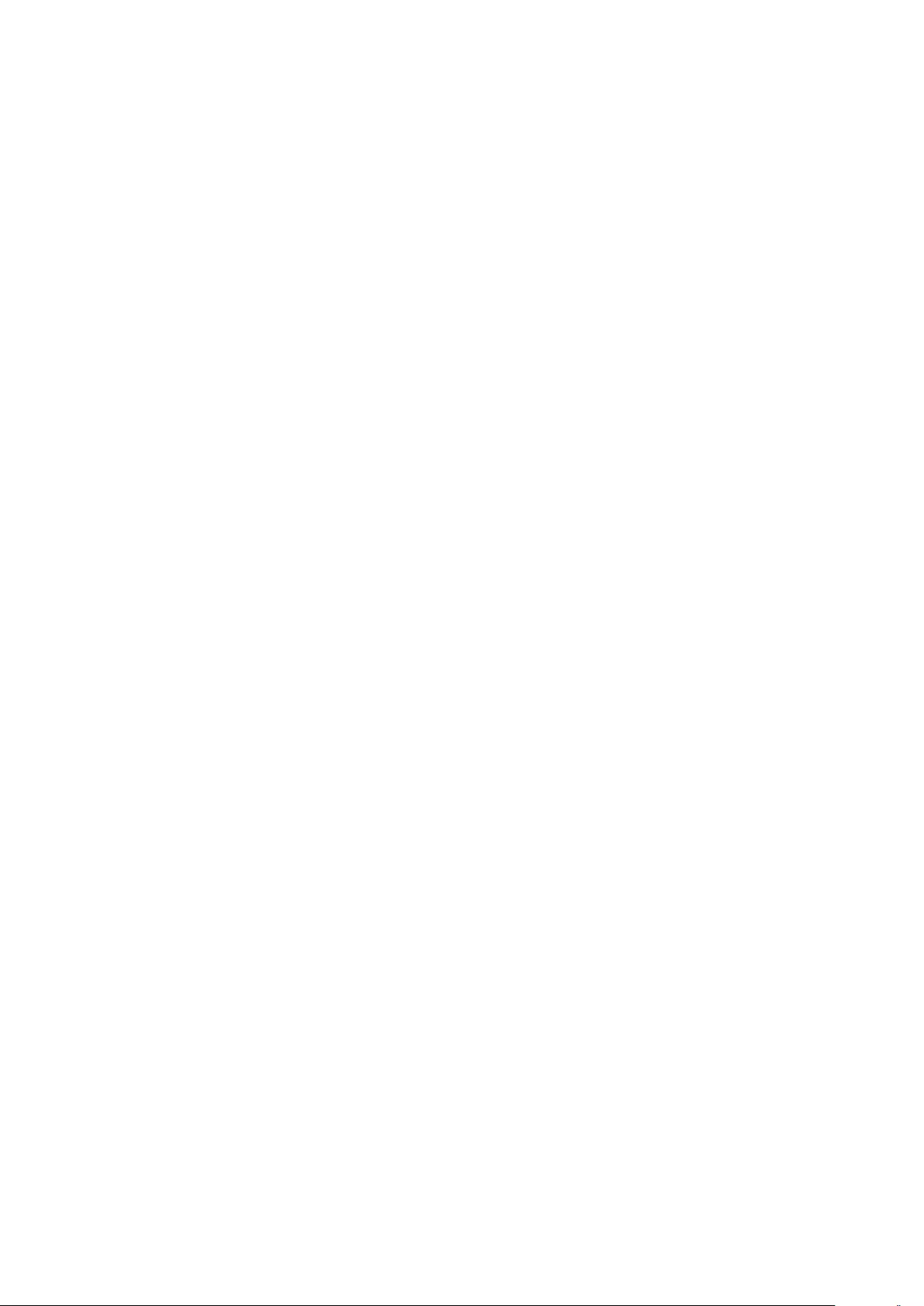
9.3 Power Amplifiers
One amplifier per loudspeaker driver
Model Bang & Olufsen ICEpower AM300-X
Peak Voltage 50 V
Peak Current 20 A
Peak Power 780 W (into 3.2 Ω)
Frequency Range <2 Hz – >100 kHz (+0 dB, - 3 dB)
THD+N 0.02% (20 Hz – 20 kHz, 100 mW - 300 W, 4 Ω, AES17)
Features DualLoop3 - ICEpower’s third-generation Class-D topology
9.4 Loudspeaker Drivers
9.4.1 Tweeter
Model Tymphany DX19TD07-08
Number 1
Nominal Impedance 8 Ω
Effective Diameter 19 mm
Features KFU membrane
Magnetic fluid
9.4.2 Midranges
Model Tymphany T04-2C0041001
Number 3
Nominal Impedance 4 Ω
Effective Diameter 87 mm
Features Kevlar reinforced paper membrane
9.4.3 Woofers
Model Tymphany T04-2B0101001
Number 3
Nominal Impedance 3 Ω
Effective Diameter 211 mm
Features EICAW voice coil wire
Rubber surround
9.5 Digital Power Link
Technology Audio Video Bridge (AVB)
Sampling Rate 192 kHz (fixed)
Bit depth 24
Features Includes proprietary B&O data channels for inter-loudspeaker communication
34
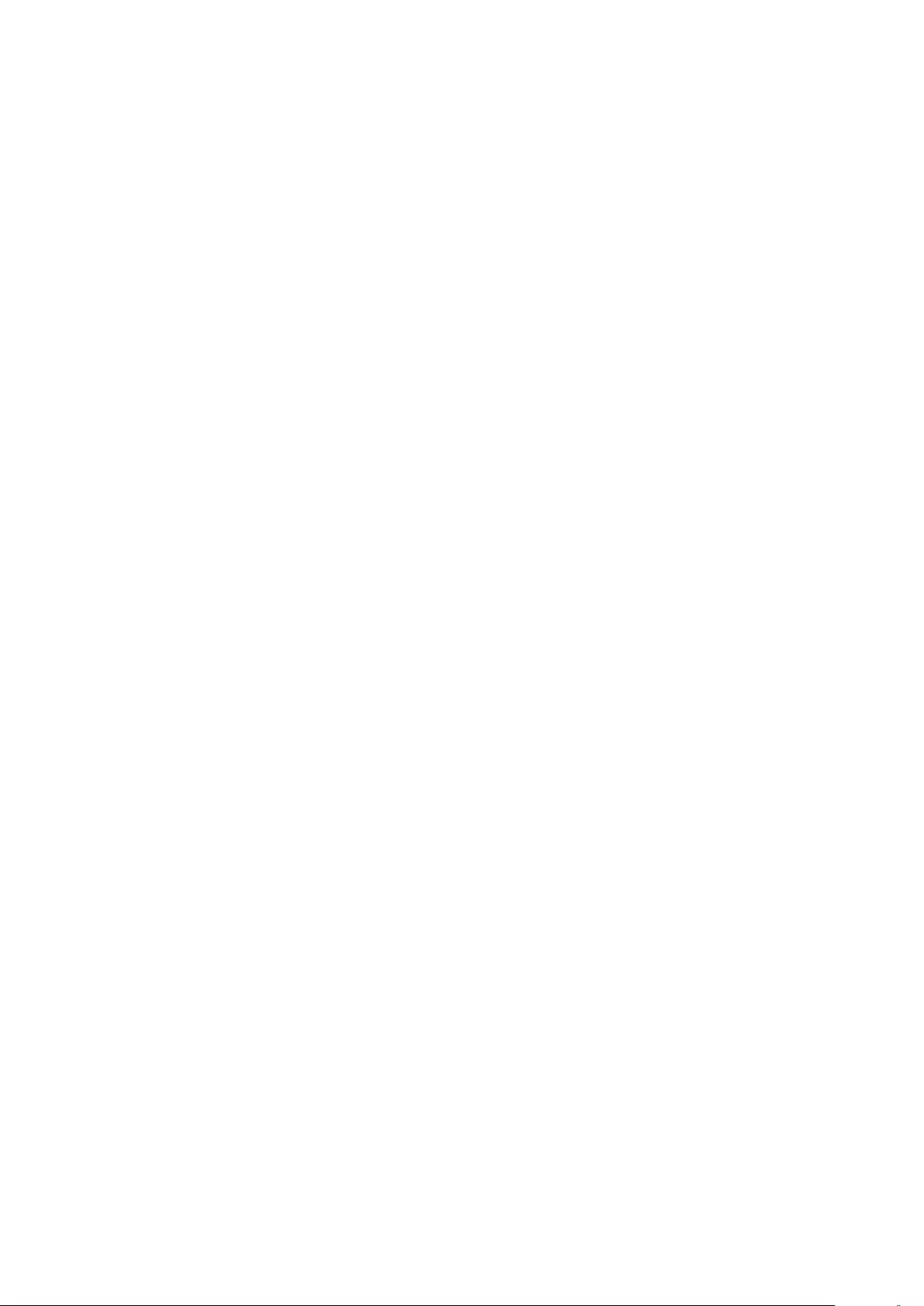
FAQ
10.1 Multichannel system
setup
In cases where you use more than one
pair of BeoLab 50’s in a configuration,
there are some recommendations that
should be followed in order to facilitate
daily use.
10.1.1 Bang & Olufsen
television as source
As described in Section 4.10.0.1, a
current Bang & Olufsen television can
automatically switch BeoLab 50
Presets as part of the Speaker Group
function. However, it should be noted
that a given Speaker Group in the
television sends only one Speaker
Preset value on its Power Link outputs
to all loudspeakers connected to the
television. This means that the preset
identification numbers in all BeoLab
50’s must match for a given
configuration corresponding to a
Speaker Group in the television.
10.1.2 Third-party device as
source
When using a third-party multichannel
device as a source for more than one
pair of BeoLab 50’s, each Master-Slave
pair of loudspeakers should be
configured correctly for a given source.
The resulting parameters should be
saved to a Preset that is then triggered
by the appropriate input. See Section
Automating Preset Selection for more
information.
10.2 Does BeoLab 50 support
DSD?
DSD and DSD over PCM (DoP) are not
currently supported by BeoLab 50. In
order to play DSD audio files, it is
therefore necessary to convert to PCM
in the audio player before sending the
signal to the loudspeakers.
Note that, since the BeoLab 50 audio
signal path contains a significant
amount of digital signal processing
(DSP) which is performed on linear PCM
signals, a conversion of DSD to PCM is
required somewhere in the audio
chain. Placing this conversion process
ahead of the loudspeakers’ inputs
gives the user the option to choose his
or her preferred filter for the process.
10.3 Does BeoLab 50 support
DXD?
DXD is not currently supported by
BeoLab 50, since its digital inputs will
not operate at sampling rates above
216 kHz.
In order to play DXD files on the
BeoLab 50, the audio signal will either
have to be downsampled to 192 kHz
(maximum) or converted to analogue
in advance of sending the signals to
the loudspeakers’ inputs.
10.4 Why does the BeoLab 50
sound “different” when I
switch to watching
television?
Some features of the BeoLab 50 are
disabled when they are connected to
current Bang & Olufsen sources. This is
to ensure that similar audio processing
is not performed twice. There are
cases, however, where although two
processes are similar, they are not
identical. For example, it may be the
case that the bass or treble
adjustments in the BeoLab 50 do not
have the same frequency responses as
those in the audio source. For more
information about this, please see
Section 12.1.
It may also be the case that the
adjustment of some of these
processors are different in the
loudspeakers and the source. For
example, if the bass is increased in the
loudspeakers, and then disabled
because the Power Link input is
chosen, there will be a resultant
change in timbre of the loudspeakers.
There may also be instances where a
Bang & Olufsen source automatically
changes the latency mode of the
BeoLab 50’s in order to preserve lip
sync or synchronisation with multiroom
systems. This will also have a
potentially audible effect on the audio
quality of the loudspeakers.
35
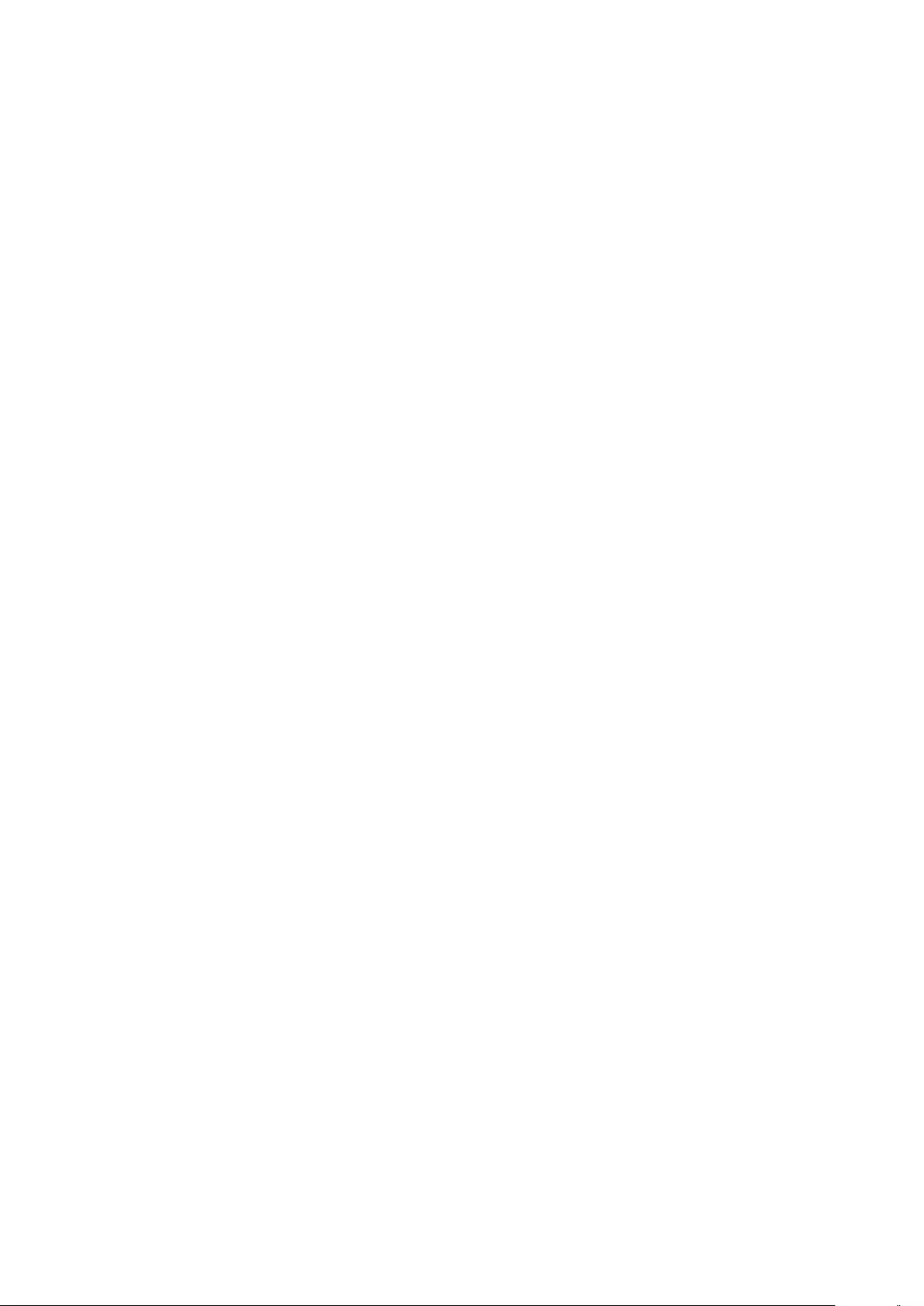
Setup “Tips and Tricks”
Setting Speaker Levels
Although it is possible to set Speaker
Levels using an SPL meter, this can, in
some cases, be improved by making
small final adjustments “by ear” while
listening to music instead. While sitting
in the preferred location, play a track
with a solid centre phantom image
(“Tom’s Diner” by Suzanne Vega is a
good choice) and adjust one of the
Speaker Level values to place the
centre image in the correct location.
This should be done after the Speaker
Distances have been adjusted.
ARC vs. Beam Width and Beam
Direction
The Active Room Compensation
algorithm calculates a custom filter for
the Beam Width, Beam Direction, and
Latency Mode of the preset. When
editing a preset, it is wise to turn off
the ARC filters while switching between
different Beam Widths, Beam
Directions, and Latency Modes in order
to avoid waiting for this calculation to
be performed. When the appropriate
settings have been chosen, then go
back to your ARC setup and enable the
filters.
iOS settings during setup
In some cases, particularly when
making ARC measurements, it may be
helpful to change the Settings of your
iOS device to ensure that it does not
“sleep”, since this can cause it to lose
communication with the BeoLab 50’s
Speaker Distances for larger
listening areas
When measuring the Speaker
Distances for larger listening areas
(with more than one person), it is
sometimes better to measure the
distance from a given loudspeaker to
the closest listener. This is particularly
true in multichannel systems.
For a “Party Mode” where there is no
single listening position (either due to
the size of the crowd, or the fact that
the listeners are moving throughout
the space), it is usually better to set
the Speaker Distances and Speaker
Levels to the matching value (e.g. 1.0
m and 0.0 dB).
Multichannel systems with more
than 2 BeoLab 50’s
In cases where two or more pairs of
BeoLab 50’s are used in a multichannel
system, then care should be taken to
ensure that the Preset numbers for the
different pairs of loudspeakers match
appropriately. This is because the
BeoVision television sends only one
Speaker Preset value to all Power Link
and Wireless Power Link outputs.
Background noise during ARC
measurements
It is important to ensure that there is
as little extraneous noise as possible
during the ARC measurement
procedure. This includes turning off air
conditioning systems or performing the
measurements during low-traffic hours,
where possible.
ARC filter calculation time
When creating an ARC filter that uses
the measurements from more than
ARC Zone, it is advisable to wait until
the first calculation is done before
including the second Zone.
36
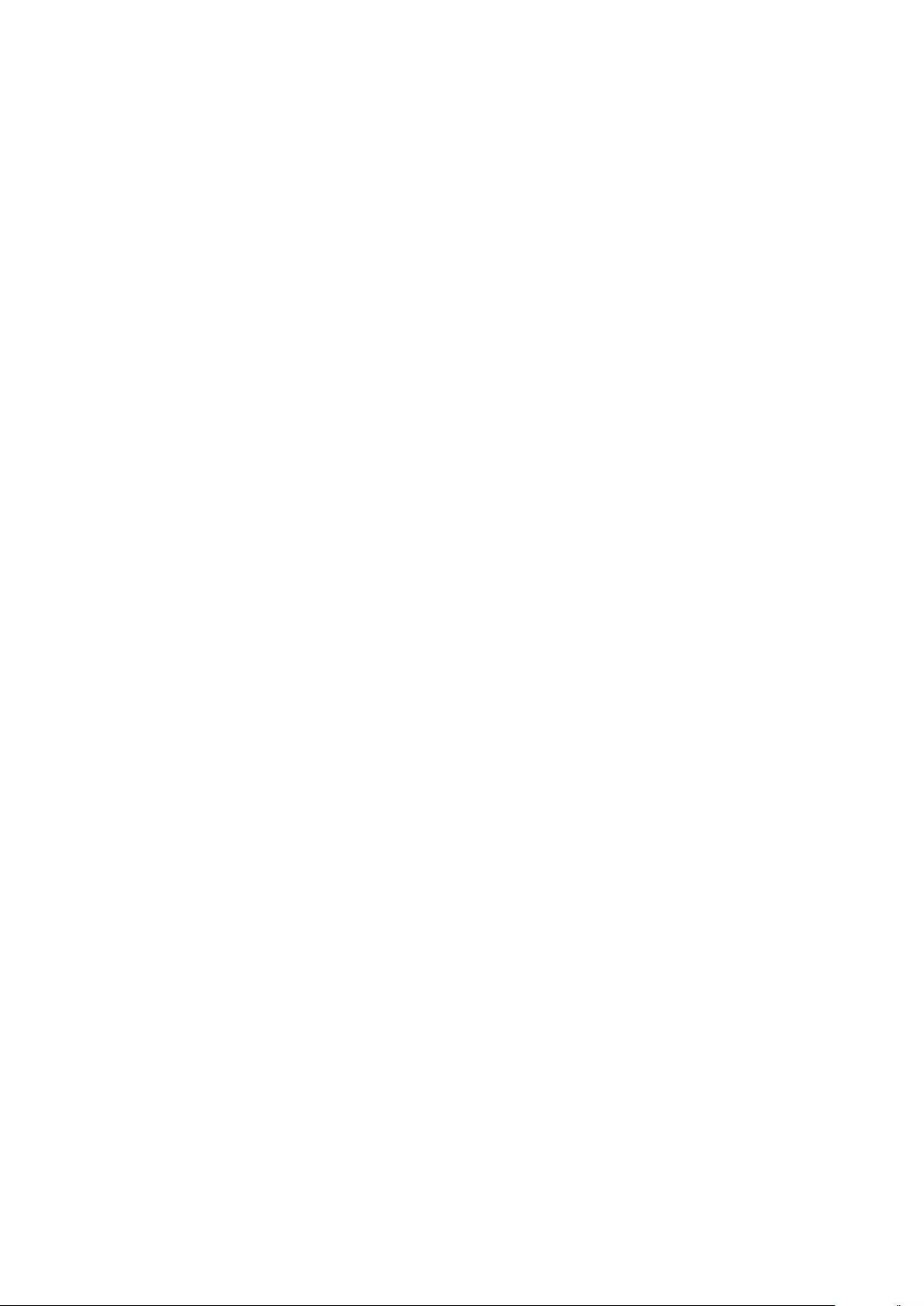
Troubleshooting
12.1 Some features in the
BeoLab 50 interface are
disabled
When connected to many Bang &
Olufsen sources via Power Link or
Wireless Power Link, some features in
the BeoLab 50 may be disabled. This is
to avoid errors such as mis-calibration
of the volume setting with other
loudspeakers in a surround
configuration or duplication of
processing (e.g. turning up the Bass
controller twice: once in the source
and once in the loudspeaker).
12.2 Lip Sync problems
When used with an older Bang &
Olufsen television (BeoSystem 3-based
or earlier) or a third-party television,
the Latency Mode of the BeoLab 50 is
not automatically controlled by the
source. Consequently, the Latency
Mode should be set to “Low” to ensure
synchronisation with the video signal.
This can be done manually using the
interface (see section 4.9.1), or set as
the default for the preset triggered by
the audio input connected to the
television.
12.3 Echo problems
12.3.1 Multiroom audio
systems
When a BeoLab 50 is used with a
third-party multiroom system, the
loudspeaker’s Latency Mode should be
set to “Low” in order to reduce the
delay time of the BeoLab 50 to a
minimum. If the Latency Mode is set to
“High” and if it is impossible to adjust
the expected loudspeaker latency in
the multiroom system, then the
BeoLab 50’s latency will be high
enough that they appear to produce an
audible echo relative to other
loudspeakers in the system.
12.3.2 Surround Processors
When a BeoLab 50 is used with an
older Bang & Olufsen surround
processor (such as the BeoSystem 3 or
earlier devices) or a third-party
surround processor, the loudspeaker’s
Latency Mode should be set to “Low” in
order to reduce the delay time of the
BeoLab 50 to a minimum. If the
Latency Mode is set to “High” and if it
is impossible to adjust the expected
loudspeaker latency in the surround
processor, then the BeoLab 50’s
latency will be high enough that they
appear to produce an audible echo
relative to other loudspeakers in the
system.
It may be possible to “trick” some
surround processors into compensating
for BeoLab 50’s latency in High
Latency Mode by adding 34.3 m (112.5
feet) to their actual distance from the
listening position. This value
corresponds to a 100 ms latency.
When used in Low Latency Mode, 8.6
m (28.1 feet) should be added to the
actual distance from the listening
position. This value corresponds to an
25 ms latency.
12.4 Loudspeakers don’t turn
on automatically
If the BeoLab 50’s are set to recognise
the Wireless Power Link / WiSA input,
then all cabled inputs are disabled.
If a cabled source is not in the list of
signals set for auto-detection as
described in Auto-detection, then it will
not automatically turn on the
loudspeakers.
It is possible that the Detection
Threshold, described in Detection
Threshold, is set to too high a value to
detect the signal.
12.5 Loudspeakers never
shut off
12.5.1 Analogue sources
Adjust the Detection Threshold higher
as described in Detection Threshold to
a higher value to prevent it from
detecting noise on the input cable.
12.5.2 Digital sources
S/P-DIF and Optical
Ensure that the signal on the digital
connection either shuts down, or
transmits a “digital black” signal. The
BeoLab 50 detects any non-zero signal
on these digital inputs and will turn on
automatically as a result.
12.6 The BeoLab 50 interface
doesn’t work
Ensure that the Digital Power Link
cable between the Master and Slave
loudspeakers is connected.
Ensure that the loudspeakers and the
device are connected to the same
network.
12.7 Loudspeakers are
distorting
The Maximum Input Level as described
in Maximum Input Voltage may be set
to too low a value to be compatible
with the audio source.
12.8 Loudspeakers are noisy /
too quiet
If the source has a variable output
level, then the best strategy for gain
management is to increase the
source’s output level to maximum and
use the volume control of the BeoLab
50’s. This will ensure the lowest
possible noise floor of the overall
system.
It is also important to ensure that the
37
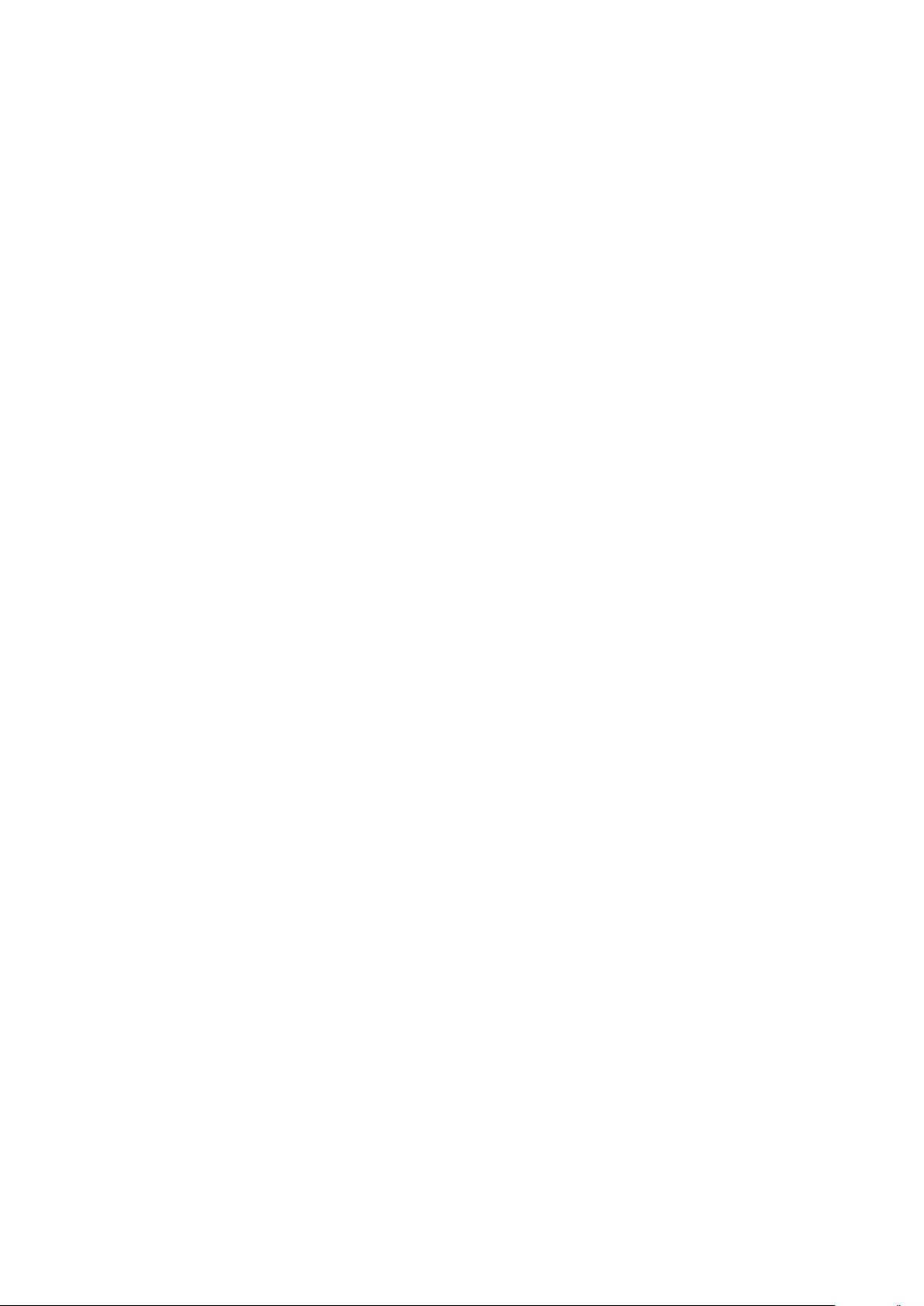
Maximum Input Level as described in
Maximum Input Voltage is set to the
correct value for the source device.
Using too high a setting will result in an
elevated noise floor.
See Input Impedance on Input
Impedance
12.9 USB Audio not working
The USB Audio input will only accept
PCM signals up to 192 kHz.
If your source is outputting DoP (also
known as DSD over PCM) or PCM
signals at higher sampling rates (e.g.
DXD at 384 kHz) there will be no audio
output from the loudspeaker.
12.10 S/P-DIF input not
working
The S/P-DIF input will only accept PCM
signals up to 192 kHz.
If your source is outputting DoP (also
known as DSD over PCM) or PCM
signals at higher sampling rates (e.g.
DXD at 384 kHz) there will be no audio
output from the loudspeaker.
If the volume control of the S/P-DIF
input using Power Link has been
enabled, then it is important to set the
priority of the S/P-DIF input higher than
that of the Power Link. If this is not
done, then the Power Link signal will
override the S/P-DIF input and the
latter will not be heard.
12.11 Optical input not
working
Note that the Optical input will not
accept sampling rates above 96 kHz
due to unreliability of an optical digital
audio connection at higher sampling
rates.
If the volume control of the Optical
input using Power Link has been
enabled, then it is important to set the
priority of the Optical input higher than
that of the Power Link. If this is not
done, then the Power Link signal will
override the Optical input and the
latter will not be heard.
12.12 Automatic switching of
inputs not behaving as
expected
If you are using the Automatic input
selection, there may be cases where
the loudspeaker does not behave as
you would intuitively expect due to the
Time out parameter of the currently
selected source. This is best explained
by giving examples.
Take the case where you are playing
audio from two sources, a CD player
connected to the S/P-DIF input and a
turntable connected to the RCA input
(via an RIAA preamplifier), and let us
assume that the S/P-DIF input has a
higher selection priority than the RCA
input. In this case, the loudspeakers
will play the CD signal. If you then
press STOP on the CD player, the
loudspeakers will not switch to the
signal on the RCA input (the turntable)
until the S/P-DIF input’s time out
duration has passed. (See Section
5.2.6 for a detailed description of the
Time-out parameter.)
This behaviour would also be true if
you were first playing a signal on the
CD player, you press STOP, and then
you start playing a signal on the
turntable. Again, until the S/P-DIF
input’s time-out duration has passed,
the signal on the RCA input (from the
turntable) will not be automatically
selected by the BeoLab 50.
38
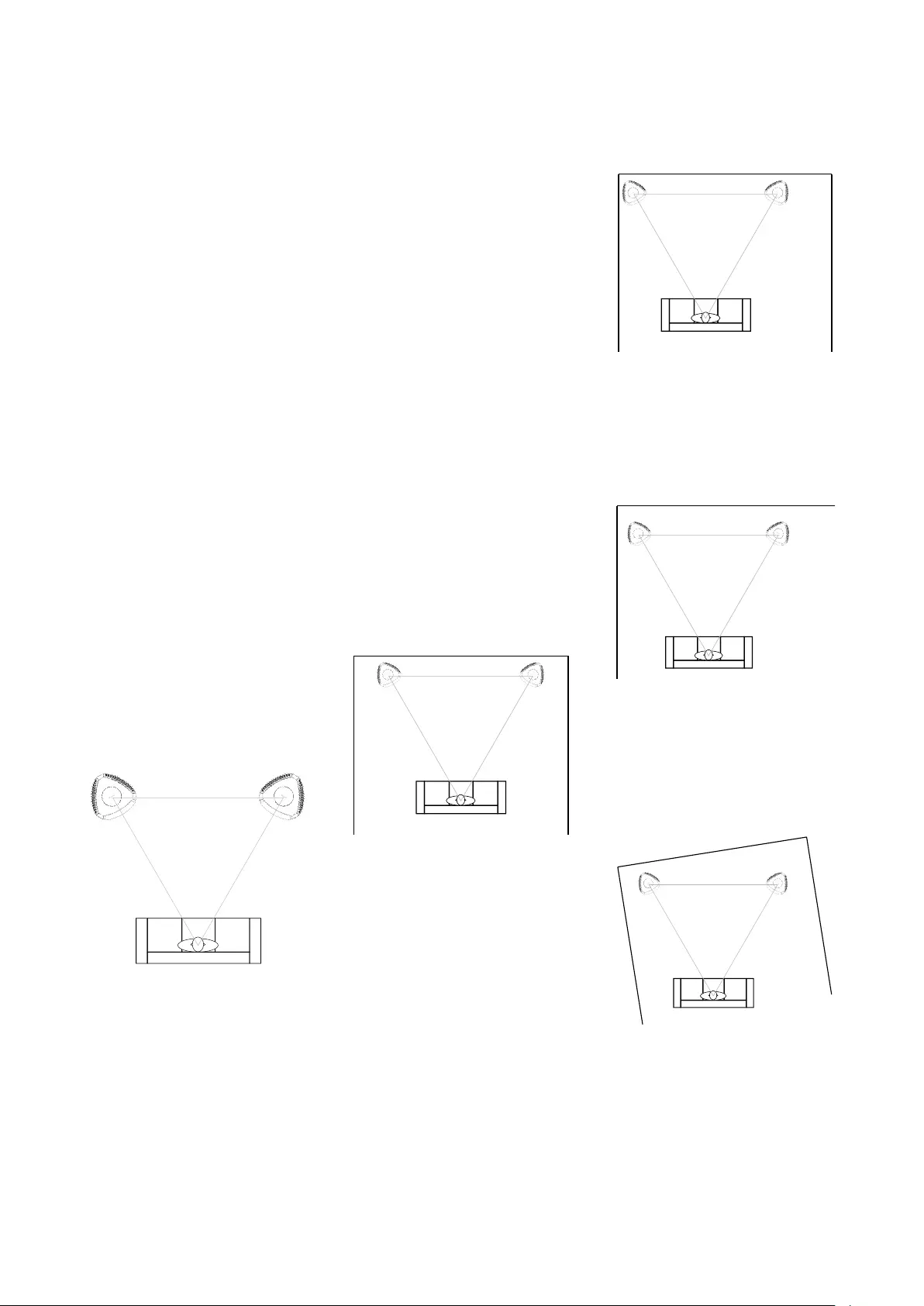
Appendix 1: Recommendations for Critical Listening
13.1 Loudspeaker
Configuration
The BeoLab 50 provides you with an
extremely wide range of parameters
that can be used to adjust the timbral
and spatial presentation of your
recordings for various listening rooms,
loudspeaker placements and listening
positions. However, it is always best to
start with an optimal configuration in
your listening room.
First consider the relationship between
the loudspeakers and the listening
position itself. The two loudspeakers
and the listening position should be the
three corners of an equilateral triangle.
This means that the distance from
each loudspeaker to the listening
position should be the same as the
distance between each loudspeaker.
This also means that the loudspeakers
will be 30◦away from the front, centre
location, directly in front of the
listening position.
Secondly, the two loudspeakers should
be “toed-in” by 30◦. This means that
they should be slightly rotated so that
they are both facing the listening
position. This is shown in Figure 13.1.
"Reflection-free Zone"
Figure 13.1: A “perfect” loudspeaker
configuration with BeoLab 50’s. Both
loudspeakers are aimed at the listening
position. The distance from the listen-
ing position to each loudspeaker is the
same as the distance between the two
loudspeakers.
If possible, the height of the listening
chair should be set so that the
listener’s ears are level with the centre
of the vertical beam, shown in Figure
??. This point is 108.6 cm above the
floor.
The next consideration is symmetry
within your listening room. It is
commonly recognised that the best
stereo imaging will be achieved if the
listening configuration (the triangle
formed with the listener and the two
loudspeakers) is placed in left–right
centre of the room. Therefore, the side
walls will both be the same distance
from the listening position, and the
loudspeakers will have the same
distance to its adjacent walls. This is to
say that the distance from the left
loudspeaker to the left wall is the same
as the distance between the right
loudspeaker and the right wall. The
distance to the front wall (behind the
loudspeakers) should be the same for
both loudspeakers, but certainly does
not have to be the same as the
distance to the side walls.
Figure 13.2: An optimal placement for
the loudspeakers with respect to adja-
cent walls. Note that the distance be-
tween each loudspeaker and its clos-
est side wall are identical, and that the
distances from the loudspeakers to the
front wall are also matched.
Figure 13.3: An less-optimal placement
for the loudspeakers with respect to ad-
jacent walls. Note that the distances
from the loudspeakers to the front wall
are matched , however, the distance be-
tween each loudspeaker and its closest
side wall are not identical.
Figure 13.4: Another example of a less-
optimal placement for the loudspeak-
ers with respect to adjacent walls. The
distances from the loudspeakers to the
front wall are matched, however, the
right loudspeaker and lacks a side wall
nearby.
Figure 13.5: An example of a worst-case
placement of loudspeakers with respect
to the listening room. No two distances
between a loudspeaker and an adjacent
wall match each other.
It should be noted that the primary
39
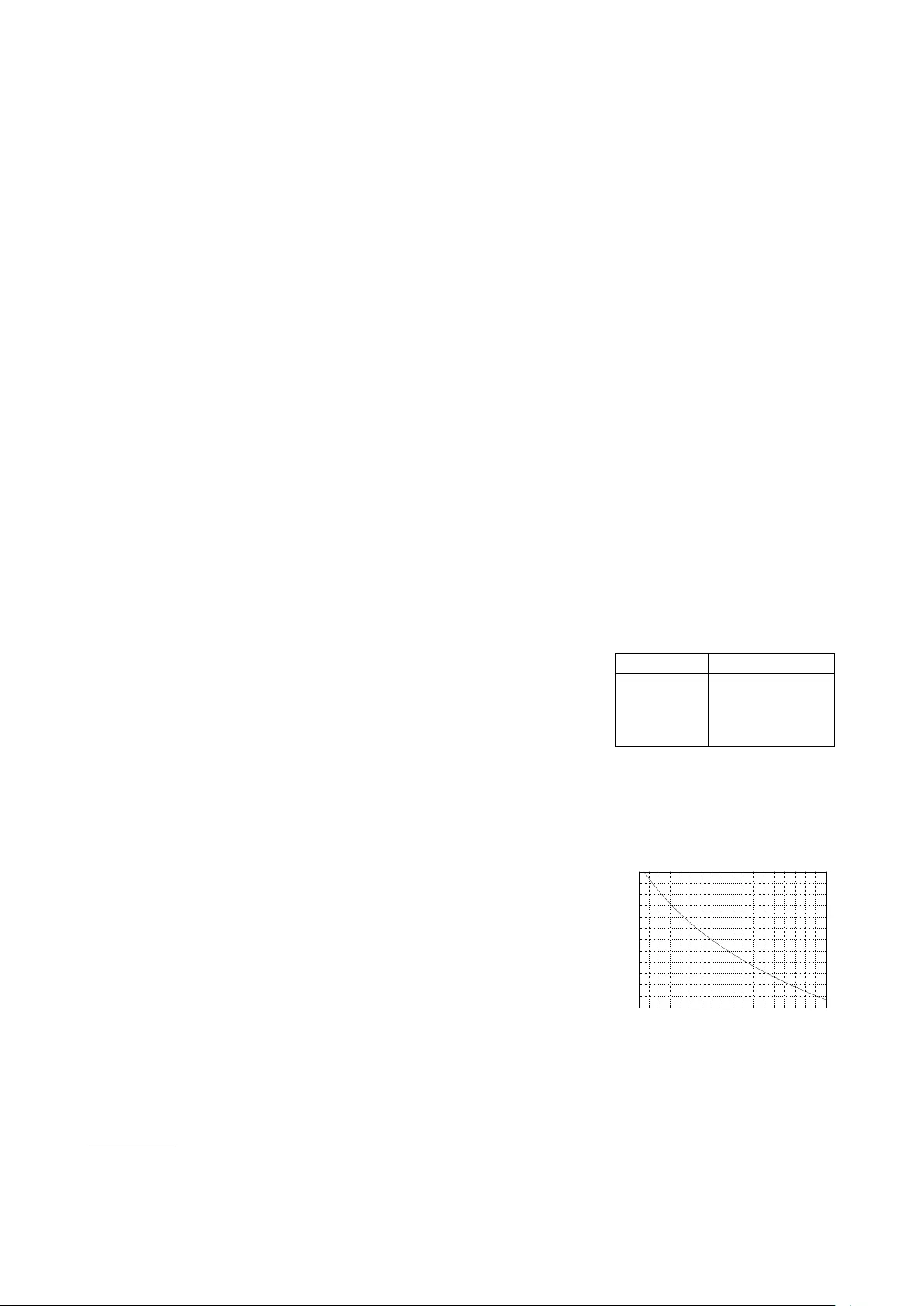
casualty of poor loudspeaker
placement in a listening room will be
the spatial representation of your
recordings. The precision and accuracy
of the stereo imaging, as well as the
sensation of envelopment from the
recording will be adversely affected by
early reflection patterns that are not
matched for the two loudspeakers.
This problem is minimised by using
BeoLab 50’s narrow beam width,
however, even this mode can benefit
from correct loudspeaker placement in
the room.
Finally, it is recommendable (but
certainly not required) that the
loudspeakers be positioned a minimum
of 1 m from the closest walls. The
Active Room Compensation algorithm
will compensate for changes in the
BeoLab 50’s timbral response caused
by adjacent boundaries. However,
placing the loudspeakers slightly
distant from reflective surfaces will
reduce these boundary effects, and
therefore also reduce the amount of
compensation that is required by the
ARC filters.
13.2 Listening Room
Acoustics
The BeoLab 50 has two features that
can overcome some detrimental
effects of the listening room’s
acoustical behaviour (Beam Width
Control and Active Room
Compensation). However, the sound of
any loudspeaker can be optimised by
improving the room’s acoustics.
One of the main acoustical problems in
listening rooms is that of room modes
or resonances. These occur because
the room behaves very much like an
organ pipe, naturally “singing” at
specific frequencies that are
determined by the dimensions of the
room. Without correct acoustical
treatment, these resonances are
almost unavoidable. It is preferred to
ensure that the resonances in the
room’s three dimensions (length,
width, and height) do not overlap each
other. This means that the better
listening rooms have complex
relationships between these three
dimensions. For example, a “worst
case” for a listening room would be a
cube, where all three dimensions are
identical, thus all resonances have the
same frequencies. A next-worst case is
one where a dimension is a multiple of
another, for example, a room that is
9m x 6m x 3m. In a best case, the
ratios of the room’s dimensions would
have non-simple values (e.g. 1 : 2.16 :
2.96 – so, as an example, 3m x 6.48m
x 8.88m).1
A second issue in many listening rooms
is that of hard, reflective surfaces –
particularly in locations where the
sound from the loudspeaker is directly
reflected to the listening position.
There are two ways to alleviate this
problem: absorption and diffusion. In
order to absorb a sound wave so that it
does not reflect off a surface, an
absorptive material such as fibreglass
insulation or acoustical foam must be
placed on the surface, or in the path
taken by the reflection. A reflection can
be diffused by making the reflective
surface irregular. For example, placing
a bookcase at the point of reflection
will help as a diffusor if the books are
arranged in random heights and
depths.
Finally, it is wise to absorb the sound
waves that would be reflected off the
floor (e.g. with carpet or a rug) and
ceiling (using absorptive ceiling tiles).
This will also help to reduce the overall
reverberation time of the room.
13.3 Source Devices
When connecting an audio source to
the BeoLab 50, there are some basic,
general rules that should be followed in
order to get the optimal performance
from your system. Note that these are
general rules – so there are exceptions.
•If possible, the source should be
connected to the BeoLab 50
using a digital audio connection.
•If the source device has a volume
control it should be disabled and
the BeoLab 50’s volume control
should be used instead
•If the source has two analogue
outputs: one volume-regulated
and the other at a fixed level, the
fixed-level output should be used
•If you are connecting a source
using a line-level analogue input,
check the source device’s
datasheet to find its maximum
output level and set the value
appropriately on the BeoLab 50
(See Maximum Input Voltage). If
the maximum output of your
device is greater than the
BeoLab 50’s maximum possible
setting (6.5 V RMS) then it is
recommendable that the source
device’s output level is reduced if
possible, either within its own
settings or using an external
attenuator. Table 13.1 and Figure
13.6 show the necessary
attenuation to reduce various
voltage levels to 6.5 V RMS.
Max Output Attenuation
7.0 V RMS -0.64 dB
9.0 V RMS -2.83 dB
11.0 V RMS -4.57 dB
13.0 V RMS -6.02 dB
Table 13.1: Examples of minimum at-
tenuation required to externally con-
vert the Maximum Output Levels from a
source device to 6.5 V RMS at the input
of the BeoLab 50.
6 8 10 12 14 16 18 20 22 24
−12
−11
−10
−9
−8
−7
−6
−5
−4
−3
−2
−1
0
Maximum Output Voltage (V rms)
Attenuation (dB)
Figure 13.6: The minimum attenuation
required to apply to a source with a
given Maximum Output level to reduce
it to 6.5 V rms in order to prevent clip-
ping the BeoLab 50 analogue inputs.
1See “Room dimensions for small listening rooms” by Dr. Trevor Cox for a good introduction to this topic.
40
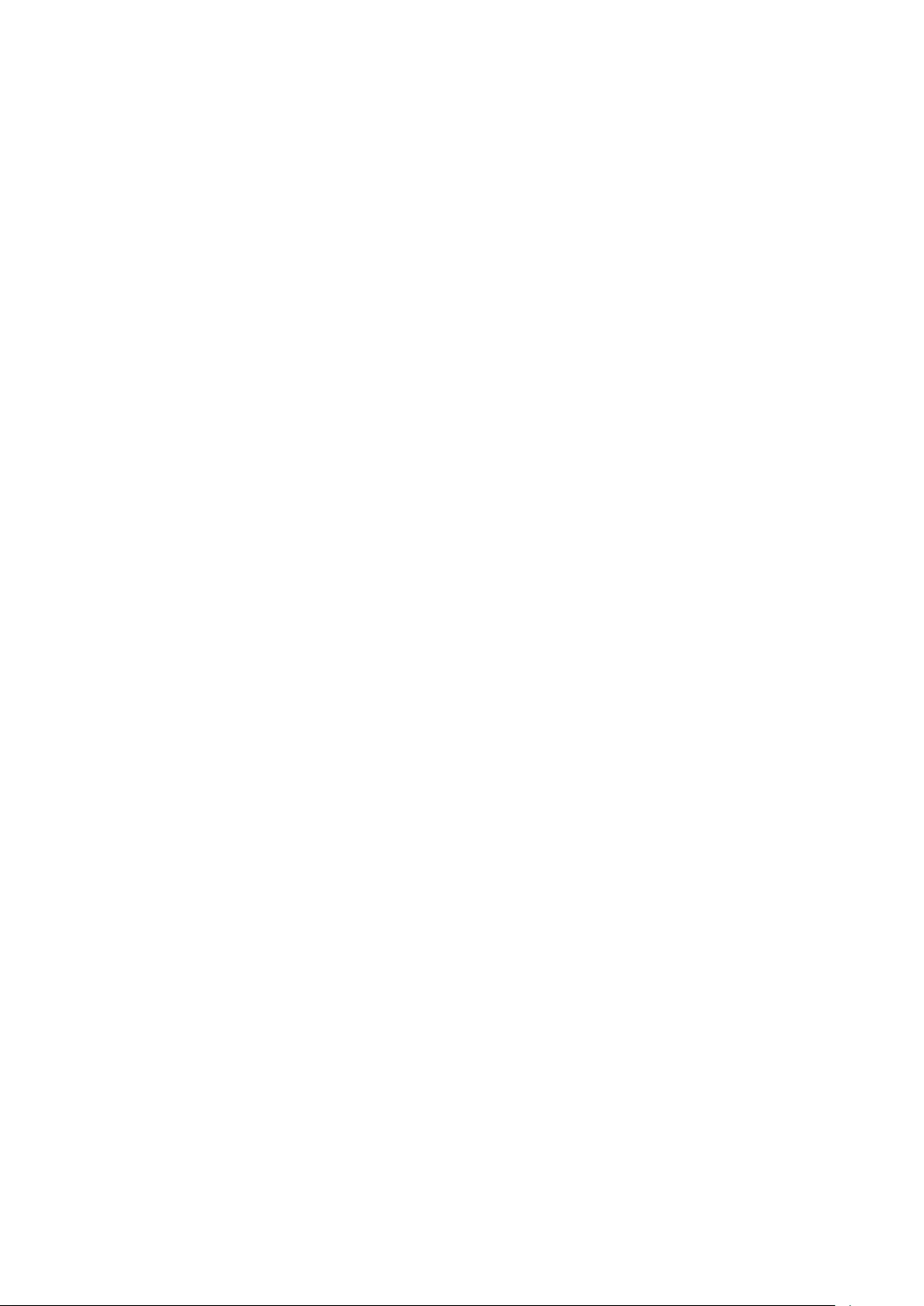
13.4 Cable recommendations
There are innumerable beliefs and
opinions, both founded and unfounded,
regarding cables used for connecting
audio devices. The following is a small
set of recommendations that are based
on common practices for wiring
professional audio systems such as are
found in recording and mastering
studios. Decisions regarding the
specific the brand or construction of
the cables used for connecting BeoLab
50 are left to the reader’s preferences.
13.4.1 Analogue cables
In order to ensure that the noise floor
of analogue sources is as low as
possible, the following guidelines are
recommended:
Use cables with good shielding (or
screening) to reduce RF (Radio
Frequency) interference on the audio
signals from external sources.
Avoid ground loops when connecting
audio devices to each other.
In order to reduce magnetic inductance
of interference (typically 50 Hz or 60
Hz “hum”) from power cables on the
audio inputs, it is also good practice to
physically separate signal cables and
mains cables as much as possible. In
cases where these cables must cross
each other, it is recommended that
they cross at a 90◦angle.
For a thorough guide to installation of
high-end audio equipment, “Audio
Systems Design and Installation” by
Philip Giddings is highly recommended.
Although this is book intended for
installation of audio devices in
recording and mastering studios, the
practices and recommendations
detailed therein are also applicable to
consumer-level audio equipment.
13.4.2 Optical cables
It is recommended that high-quality
optical cables are used for the BeoLab
50, particularly for longer cable runs.
This is due to the fact that there is
attenuation (dimming or loss of light
intensity) of the optical signal on the
plastic or glass fibre in the cable. This
attenuation is proportional (in dB) to
the length of the cable. Therefore, in
order to ensure that the optical
receiver on the BeoLab 50 has an
adequate signal at its input, the light
attenuation on the cable should be
minimised either by using short cables
or high-quality optical fibre.
Traditionally, many people have
claimed that optical digital signals are
less reliable than electrical connections
(such as the AES/EBU and S/P-DIF
protocols) due to higher levels of jitter
caused by the limitations of the rise
and fall time of the LED in the
transmitter. The BeoLab 50 uses a
very-high-quality sampling rate
converter at its input for all digital
signals which attenuates the jitter of
incoming sources, thereby reducing
this concern considerably.
13.4.3 S/P-DIF cables
When connecting a source to BeoLab
50’s S/P-DIF input, it is recommended
that a cable with a 75 Ωimpedance is
used. This will ensure that there are no
reflections of the signal on the cable
which may increase the level of jitter at
the input of the BeoLab 50. Note that
this recommendation is particularly
true for longer cable runs. It should,
however, be stated that the sampling
rate converter at the digital inputs of
the BeoLab 50 is very effective at
attenuating jitter artefacts caused
either by the signal source or problems
in the cabling.
13.5 AC mains cables
It is highly recommended that an
additional device used to filter the AC
power from the mains (sometimes
called an “audiophile mains filter” or
“power purifier”, for example) not be
used with the BeoLab 50. This is
because the internal power supply of
the BeoLab 50 has a custom-designed
filter that reduces noise on its AC
mains input. This filter has been
optimised for the time-variant current
demands of the BeoLab 50, making a
generic external filter redundant (at
best) or detrimental (at worst) to the
performance of the loudspeaker.
Similarly, it is unnecessary to use a
so-called “exotic” or “audiophile”
mains cable for the BeoLab 50.
41

Appendix 2: Introduction to Parametric Equalisers
Almost all sound systems offer bass
and treble adjustments for the sound –
these are basically coarse versions of a
more general tool called an equaliser
that is often used in recording studios.
Once upon a time, if you made a
long-distance phone call, there was an
actual physical connection made
between the wire running out of your
telephone and the telephone at the
other end of the line. This caused a big
problem in signal quality because a lot
of high-frequency components of the
signal would get attenuated along the
way due to losses in the wiring.
Consequently, booster circuits were
made to help make the relative levels
of the various frequencies more equal.
As a result, these circuits became
known as equalisers. Nowadays, of
course, we don’t need to use
equalisers to fix the quality of
long-distance phone calls (mostly
because the communication paths use
digital encoding instead of analogue
transmission), but we do use them to
customise the relative balance of
various frequencies in an audio signal.
This happens most often in a recording
studio, but equalisers can be a great
personalisation tool in a playback
system in the home.
The two main reasons for using
equalisation in a playback system such
as the BeoLab 50’s are personal
preference and compensation for the
effects of the listening room’s
acoustical behaviour.
Equalisers are typically comprised of a
collection of filters, each of which has
up to 4 “handles” or “parameters” that
can be manipulated by the user. These
parameters are
•Filter Type
•Gain
•Centre Frequency
•Q
14.1 Filter Type
The Filter Type will let you decide the
relative levels of signals at frequencies
within the band that you’re affecting.
Although there are up to 7 different
types of filters that can be found in
professional parametric equalisers, the
BeoLab 50 contains the three
most-used of these:
•low-shelving Filter
•high-shelving Filter
•Peaking Filter
14.1.1 low-shelving Filter
In theory, a low-shelving Filter affects
gain of all frequencies below the centre
frequency by the same amount. In
reality, there is a band around the
centre frequency where the filter
transitions between a gain of 0 dB (no
change in the signal) and the gain of
the affected frequency band.
10 100 1,000 10,000
−6
−4
−2
0
2
4
6
Frequency (Hz)
Gain (dB)
Figure 14.1: Example of a low-shelving
filter with a positive gain. Frequencies
below approximately 80 Hz have been
affected.
10 100 1,000 10,000
−6
−4
−2
0
2
4
6
Frequency (Hz)
Gain (dB)
Figure 14.2: Example of a low-shelving
filter with a negative gain. Frequencies
below approximately 80 Hz have been
affected.
Note that the low-shelving filters used
in the BeoLab 50 define the centre
frequency as being the frequency
where the gain is one half the
maximum (or minimum) gain of the
filter. For example, in Figure 14.1, the
gain of the filter is 6 dB. The centre
frequency is the frequency where the
gain is one-half this value or 3 dB,
which can be found at 80 Hz.
Some care should be taken when using
low-shelving filters since their affected
frequency bands extend to 0 Hz or DC.
This can cause a system to be pushed
beyond its limits in extremely low
frequency bands that are of little-to-no
consequence to the audio signal. Note,
however, that this is less of a concern
for the BeoLab 50, since it is protected
against such abuse.
14.1.2 high-shelving Filter
In theory, a high-shelving Filter affects
gain of all frequencies above the
centre frequency by the same amount.
In reality, there is a band around the
centre frequency where the filter
transitions between a gain of 0 dB (no
change in the signal) and the gain of
the affected frequency band.
42
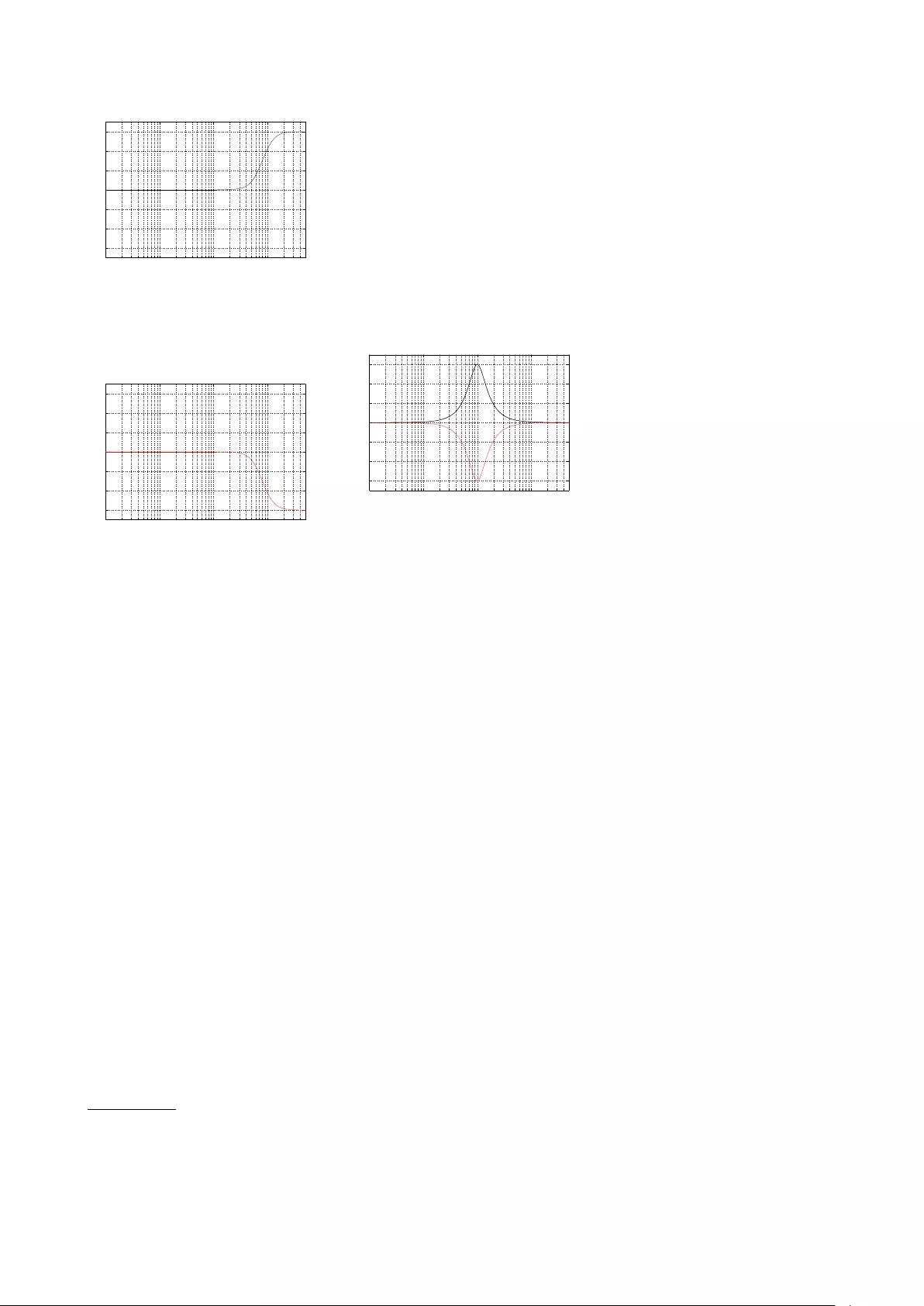
10 100 1,000 10,000
−6
−4
−2
0
2
4
6
Frequency (Hz)
Gain (dB)
Figure 14.3: Example of a high-shelving
filter with a positive gain. Frequencies
above approximately 8 kHz have been
affected.
10 100 1,000 10,000
−6
−4
−2
0
2
4
6
Frequency (Hz)
Gain (dB)
Figure 14.4: Example of a high-shelving
filter with a negative gain. Frequencies
above approximately 8 kHz have been
affected.
Note that the high-shelving filters used
in the BeoLab 50 define the centre
frequency as being the frequency
where the gain is one half the
maximum (or minimum) gain of the
filter. For example, in Figure 14.4, the
gain of the filter is -6 dB. The centre
frequency is the frequency where the
gain is one-half this value or -3 dB,
which can be found at 8 kHz.
Some care should be taken when using
high-shelving filters since their
affected frequency bands can extend
beyond the audible frequency range.
This can cause a system to be pushed
beyond its limits in extremely high
frequency bands that are of little-to-no
consequence to the audio signal.
14.1.3 Peaking Filter
Apeaking filter is used for a more local
adjustment of a frequency band. In this
case, the centre frequency of the filter
is affected most (it will have the Gain
of the filter applied to it) and adjacent
frequencies on either side are affected
less and less as you move further
away. For example, Figure 14.5 shows
the response of a peaking filter with a
centre frequency of 1 kHz and gains of
6 dB (the black curve) and -6 dB (the
red curve). As can be seen there, the
maximum effect happens at 1 kHz and
frequency bands to either side are
affected less.
10 100 1,000 10,000
−6
−4
−2
0
2
4
6
Frequency (Hz)
Gain (dB)
Figure 14.5: Example of two peaking fil-
ters. The black curve shows a filter with
a positive gain, the red curve shows the
reciprocal with a negative gain. The
centre frequency of this filter is 1 kHz.
You may notice in Figure 14.5 that the
black and red curves are symmetrical –
in other words, they are identical
except in polarity of the gain. This is a
particular type of peaking filter called a
reciprocal peak/dip filter – so-called
because these two filters, placed in
series, can be used to cancel each
other’s effects on the signal.
Note that BeoLab 50 uses reciprocal
peak/dip filters.
14.2 Gain
If you need to make all frequencies in
your audio signal louder, then you just
need to increase the volume. However,
if you want to be a little more selective
and make some frequency bands
louder (or quieter) and leave other
bands unchanged, then you’ll need an
equaliser. So, one of the important
questions to ask is “how much louder?”
or “how much quieter?” The answer to
this question is the gain of the filter –
this is the amount by which is signal is
increased or decreased in level.
The gain of an equaliser filter is almost
always given in decibels or dB1. This is
a scale based on logarithmic changes
in level. Luckily, it’s not necessary to
understand logarithms in order to have
an intuitive feel for decibels. There are
really just three things to remember:
•a gain of 0 dB is the same as
saying “no change”
•positive decibel values are
louder, negative decibel values
are quieter
•Adding approximately 6 dB to the
gain is the same as saying “two
times the level”. (Therefore,
subtracting 6 dB is half the level.)
14.3 Centre Frequency
So, the next question to answer is
“which frequency bands do you want
to affect?” This is partially defined by
the centre frequency or Fc of the filter.
This is a value that is measured in the
number of cycles per second2, labelled
Hertz or Hz.
Generally, if you want to increase (or
reduce) the level of the bass, then you
should set the centre frequency to a
low value (roughly speaking, below
125 Hz). If you want to change the
level of the high frequencies, then you
should set the centre frequency to a
high value (say, above 8 kHz).
14.4 Q
In all of the above filter types, there
are transition bands – frequency areas
where the filter’s gain is changing from
0 dB to the desired gain. Changing the
filter’s Q3allows you to alter the shape
of this transition. The lower the Q, the
smoother the transition. In both the
case of the shelving filters and the
peaking filter, this means that a wider
1The “B” is a capital because it’s named after Alexander Graham Bell.
2This is literally the number of times a loudspeaker driver will move in and out of the loudspeaker cabinet per second.
3Note that, although the term “Q” is used throughout this manual and the BeoLab 50 interface for both peaking and shelving filters, this is incorrect. To be technically correct,
the term “S” (or shelf slope) should be used for shelving filters.
43
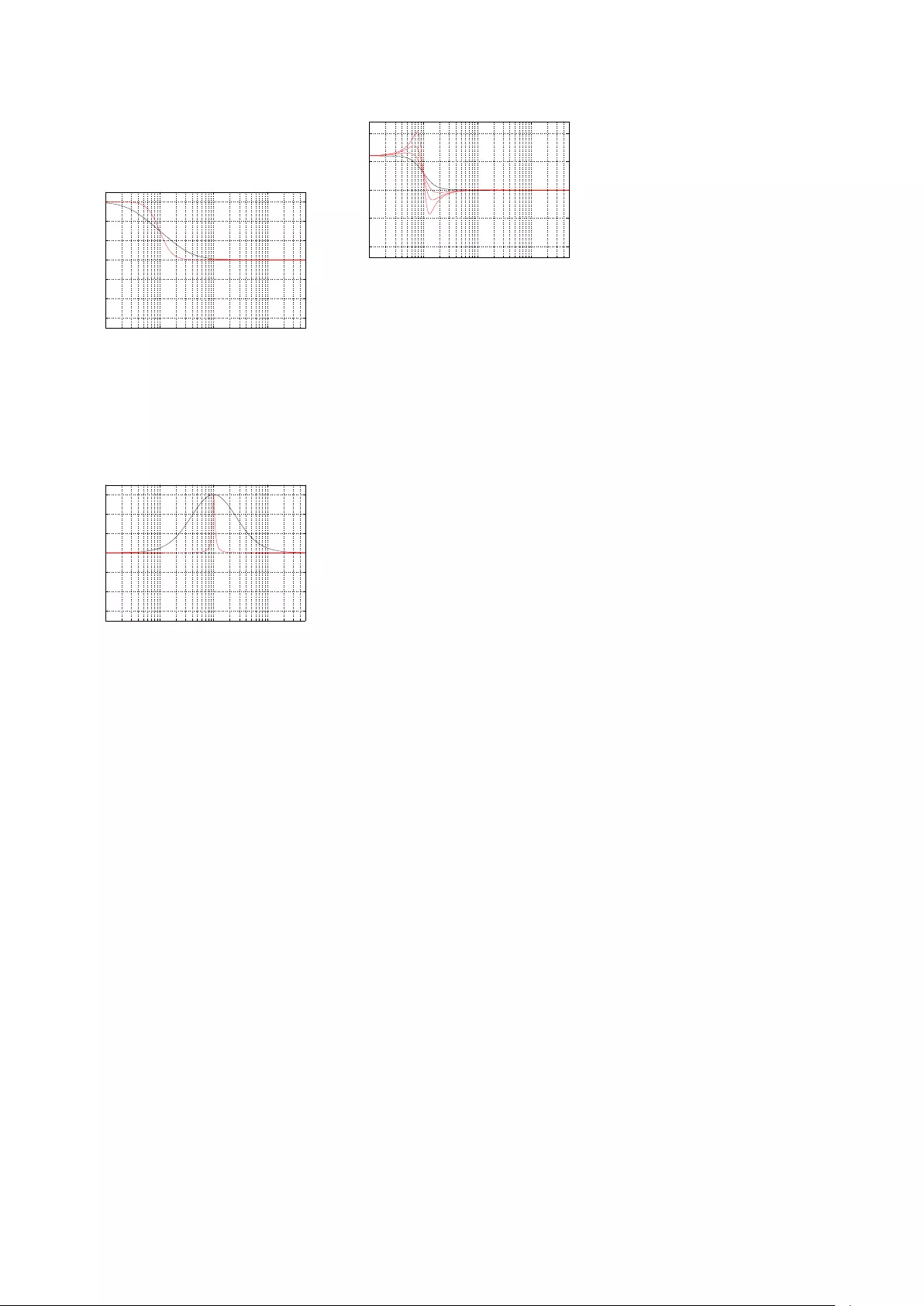
band of frequencies will be affected.
This can be seen in the examples in
Figures 14.6 and 14.7.
10 100 1,000 10,000
−6
−4
−2
0
2
4
6
Frequency (Hz)
Gain (dB)
Figure 14.6: Example of two low-
shelving filters. The black curve shows
a filter with a Q of 0.35, the red curve
shows the a filter with a Q of 1. For both
filters, the centre frequency is 1 kHz and
the gain is +6 dB.
10 100 1,000 10,000
−6
−4
−2
0
2
4
6
Frequency (Hz)
Gain (dB)
Figure 14.7: Example of two peaking fil-
ters. The black curve shows a filter with
a Q of 0.35, the red curve shows the a
filter with a Q of 8. For both filters, the
centre frequency is 1 kHz and the gain
is +6 dB.
It should be explained that the Q
parameter can cause a shelving filter
to slightly differently than a peaking
filter. When the Q of a shelving filter
exceeds a value of 1, the gain of the
filter will “overshoot” its limits. For
example, as can be seen in Figure 14.8,
a filter with a gain of 6 dB and a Q of 8
will actually have a gain of over 10 dB
and will attenuate by more than 4 dB.
This over- and undershooting of the
filter’s magnitude response is the
reason the Q of the high-shelving and
low-shelving filters in the BeoLab 50’s
parametric equaliser have been limited
to a maximum value of 1.
10 100 1,000 10,000
−10
−5
0
5
10
Frequency (Hz)
Gain (dB)
Figure 14.8: Example of low-shelving fil-
ters with a Q of more than 1. The black
curve shows a filter with a Q of 1 for ref-
erence, the red curves shows the a filter
with Q’s of 2, 4, and 8. The centre fre-
quency of this filter is 1 kHz and the gain
is +6 dB.
44

Appendix 3: The Influence of Listening Room Acoustics on Loudspeakers
A room comprised of large flat
reflective surfaces with little acoustical
absorption has a very different
acoustical behaviour from a recording
or mastering studio where the final
decisions about various aspects of a
recording are made. Consequently,
this must have an effect on a listener’s
perception of a recording played
through a pair (assuming stereo
reproduction) of loudspeakers in that
room. The initial question to be asked
is “what, exactly, are the expected
effects of the room’s acoustical
behaviour in such a case?” The second
is “if the room has too much of an
effect, how can I improve the situation
(e.g. by adding absorption or changing
the physical configuration of the
system in the room)?” The third, and
possibly final question is “how can a
loudspeaker compensate (or at least
account) for these effects?”
The effect a room’s acoustical
behaviour has on a loudspeaker’s
sound can, at a simple level, be
considered under three general
headings:
•Early Reflections
•Room Modes
•Reverberation
15.1 Early Reflections
Early reflections, from sidewalls and
the floor and ceiling, have an influence
on both the timbre (tone colour) and
the spatial characteristics of a stereo
reproduction system. We will only
discuss the timbral effects in this
article.
Figure 15.1: The sound arriving at a lis-
tener from a loudspeaker in a room with
only one wall. Note that the sound ar-
rives from two directions – the first is
directly from the loudspeaker (in red).
The second is a “first reflection” off the
wall (in blue).
Let’s start by assuming that you have
a loudspeaker that has a magnitude
response that is perfectly flat – at least
from 20 Hz to 20 kHz. We will also
assume that it has that response
regardless of which direction you
measure it in – in other words, it’s a
perfectly omnidirectional loudspeaker.
The question is, “what effect does the
wall reflection have on the measured
response of the loudspeaker?”
Very generally speaking, the answer is
that you will get a higher level at some
frequencies (because the direct sound
and the reflection add constructively
and reinforce each other) and you will
get a lower level at other frequencies
(because the direct sound and the
reflection work against each other and
“cancel each other out”). What is
potentially interesting is that the
frequencies that add and the
frequencies that cancel alternate as
you go up the frequency range. So the
total result looks like a comb (as in a
comb that you use to comb your hair,
if, unlike me, you have hair to comb).
For example, take a look at Figure 15.2.
10 100 1,000 10,000
−20
−15
−10
−5
0
Frequency (Hz)
Gain (dB)
Figure 15.2: Distance to loudspeaker =
2 m. Distance to wall = 1 m. Wall is per-
fectly reflective and the loudspeaker is
perfectly omnidirectional. The red line
is the magnitude response of the direct
sound. The blue line is the magnitude
response of the reflected sound. The
black line is the magnitude response of
the combination.
You can see that, at the very low end,
the reflection boosts the level of the
loudspeaker by a approximately 5 dB
(or almost two times the level) at the
listening position. However, as you go
up in frequency, the total level drops to
about 15 dB less before it starts rising
again. As you go up in frequency, the
level goes up and down. This
alternation actually happens at a
regular frequency spacing (e.g. a notch
at multiples of 200 Hz) but it doesn’t
look regular because the X-axis of the
plot is logarithmic (which better
represents how we hear differences in
frequency).
What happens if we move the wall
further away? Well, two things will
happen. The first is that the reflection
will be quieter, so the peaks and
notches won’t be as pronounced. The
second is that the spacing of the peaks
and notches in frequency will get
closer together. In other words, the
effect starts at a lower frequency.
For example, take a look at Figure 15.3.
45
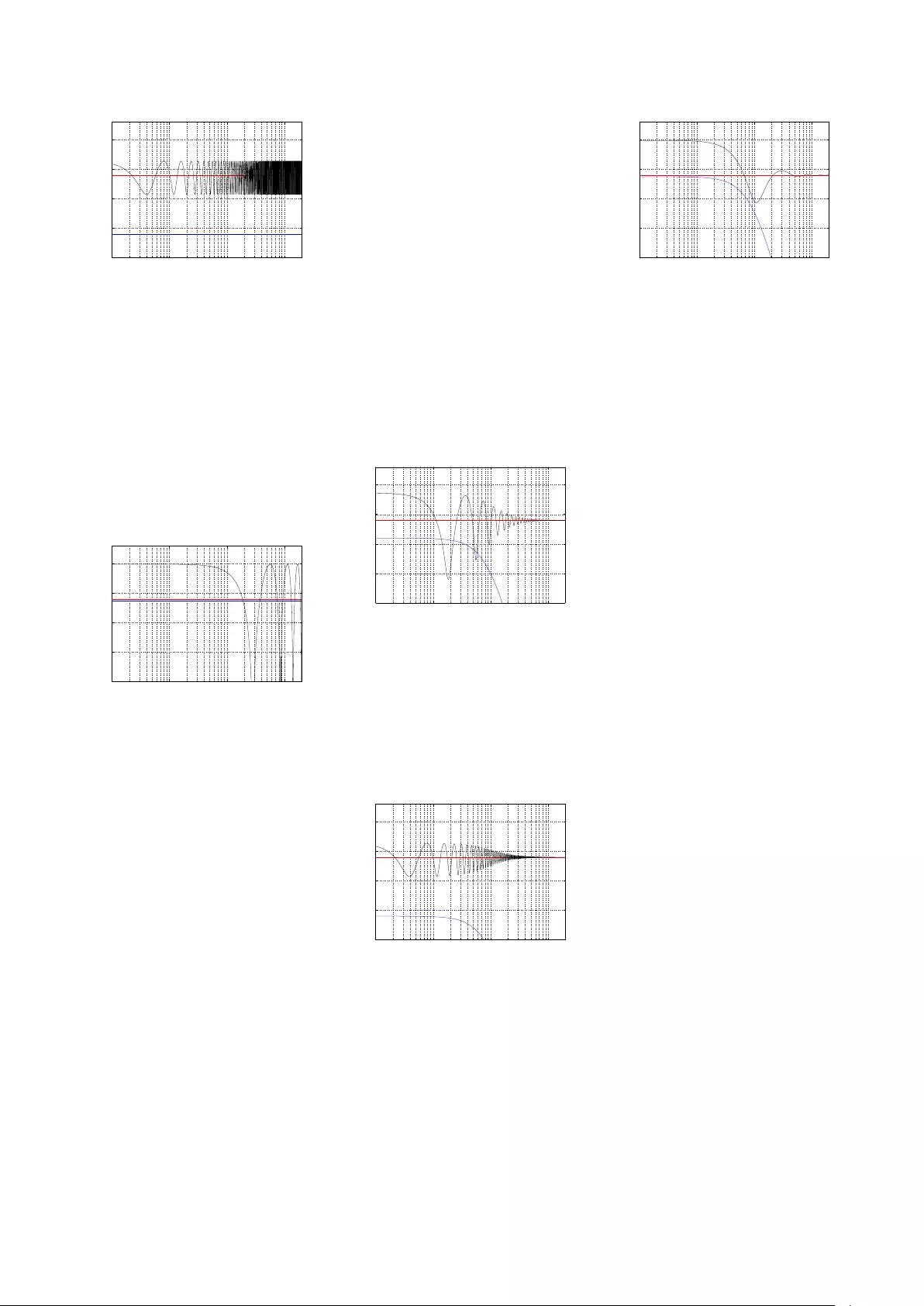
10 100 1,000 10,000
−20
−15
−10
−5
0
Frequency (Hz)
Gain (dB)
Figure 15.3: Distance to loudspeaker =
2 m. Distance to wall = 3 m. Wall is per-
fectly reflective and the loudspeaker is
perfectly omnidirectional. The red line
is the magnitude response of the direct
sound. The blue line is the magnitude
response of the reflected sound. The
black line is the magnitude response of
the combination.
Conversely, if we move the wall closer,
we do the opposite (the problem gets
worse, but at a higher frequency), as
can be seen in Figure 15.4.
10 100 1,000 10,000
−20
−15
−10
−5
0
Frequency (Hz)
Gain (dB)
Figure 15.4: Distance to loudspeaker =
2 m. Distance to wall = 0.25 m. Wall is
perfectly reflective and the loudspeaker
is perfectly omnidirectional. The red
line is the magnitude response of the
direct sound. The blue line is the mag-
nitude response of the reflected sound.
The black line is the magnitude re-
sponse of the combination.
So, if you have a room with only one
wall which is perfectly reflective, and
you have a perfectly omnidirectional
loudspeaker, then you can see that
your best option is to either put the
loudspeaker (and yourself) very far or
very close to the wall. That way the
artefacts caused by the reflection are
either too quiet to do any damage, or
have an effect that starts at too high a
frequency for you to care. Then again,
most room have more than one wall,
the walls are not perfectly reflective,
and the loudspeaker is not perfectly
omnidirectional.
So, what happens in the case where
the loudspeaker is more directional or
you have some absorption (better
known as “fuzzy stuff”) on your walls?
Well, either of these cases will have
basically the same effect in most cases
since loudspeakers are typically more
directional at high frequencies – so you
get less high end directed towards the
wall. Alternatively, fuzzy stuff tends to
soak up high frequencies. So, in either
of these two cases, you’ll get less high
end in the reflection. Let’s simulate
this by putting a low pass filter on the
reflection, as shown in Figure 15.5,
15.6 and 15.7 which have identical
distances as the simulations in Figures
15.2,15.3, and 15.4 – for comparison.
10 100 1,000 10,000
−20
−15
−10
−5
0
Frequency (Hz)
Gain (dB)
Figure 15.5: Distance to loudspeaker =
2 m. Distance to wall = 1 m. Wall is ab-
sorptive and/or the loudspeaker is direc-
tional at high frequencies. The red line
is the magnitude response of the direct
sound. The blue line is the magnitude
response of the reflected sound. The
black line is the magnitude response of
the combination.
10 100 1,000 10,000
−20
−15
−10
−5
0
Frequency (Hz)
Gain (dB)
Figure 15.6: Distance to loudspeaker =
2 m. Distance to wall = 3 m. Wall
is absorptive and/or the loudspeakers
is directional at high frequencies. The
red line is the magnitude response of
the direct sound. The blue line is the
magnitude response of the reflected
sound. The black line is the magnitude
response of the combination.
10 100 1,000 10,000
−20
−15
−10
−5
0
Frequency (Hz)
Gain (dB)
Figure 15.7: Distance to loudspeaker =
2 m. Distance to wall = 0.25 m. Wall
is absorptive and/or the loudspeaker is
directional at high frequencies. The red
line is the magnitude response of the di-
rect sound. The blue line is the mag-
nitude response of the reflected sound.
The black line is the magnitude re-
sponse of the combination.
What you can see in all three of the
previous plots is that, as the high
frequency content of the reflection
disappears, there is less and less effect
on the total. The bottom plot is
basically a proof of the age-old rule of
thumb that says that, if you put a
loudspeaker next to a wall, you’ll get
more bass than if it’s farther from the
wall. Since there is not much high
frequency energy radiated from the
rear of most loudspeakers, Figure 15.7
is a pretty good general representation
of what happens when a loudspeaker is
placed close to a wall. Of course, the
exact behaviour of the directivity of the
loudspeaker will be different – but the
general shape of the total curve will be
pretty similar to what you see there.
So, the end conclusion of all of this is
that, in order to reduce undesirable
artefacts caused by a wall reflection,
you can do any combination of the
following:
•move the loudspeaker very close
to the wall
•move the loudspeaker farther
front the wall
•sit very close to the wall
•sit farther away from the wall
•put absorption on the wall
However, there is one interesting
effect that sits on top of all of this –
that is the fact that what you’ll see in a
46
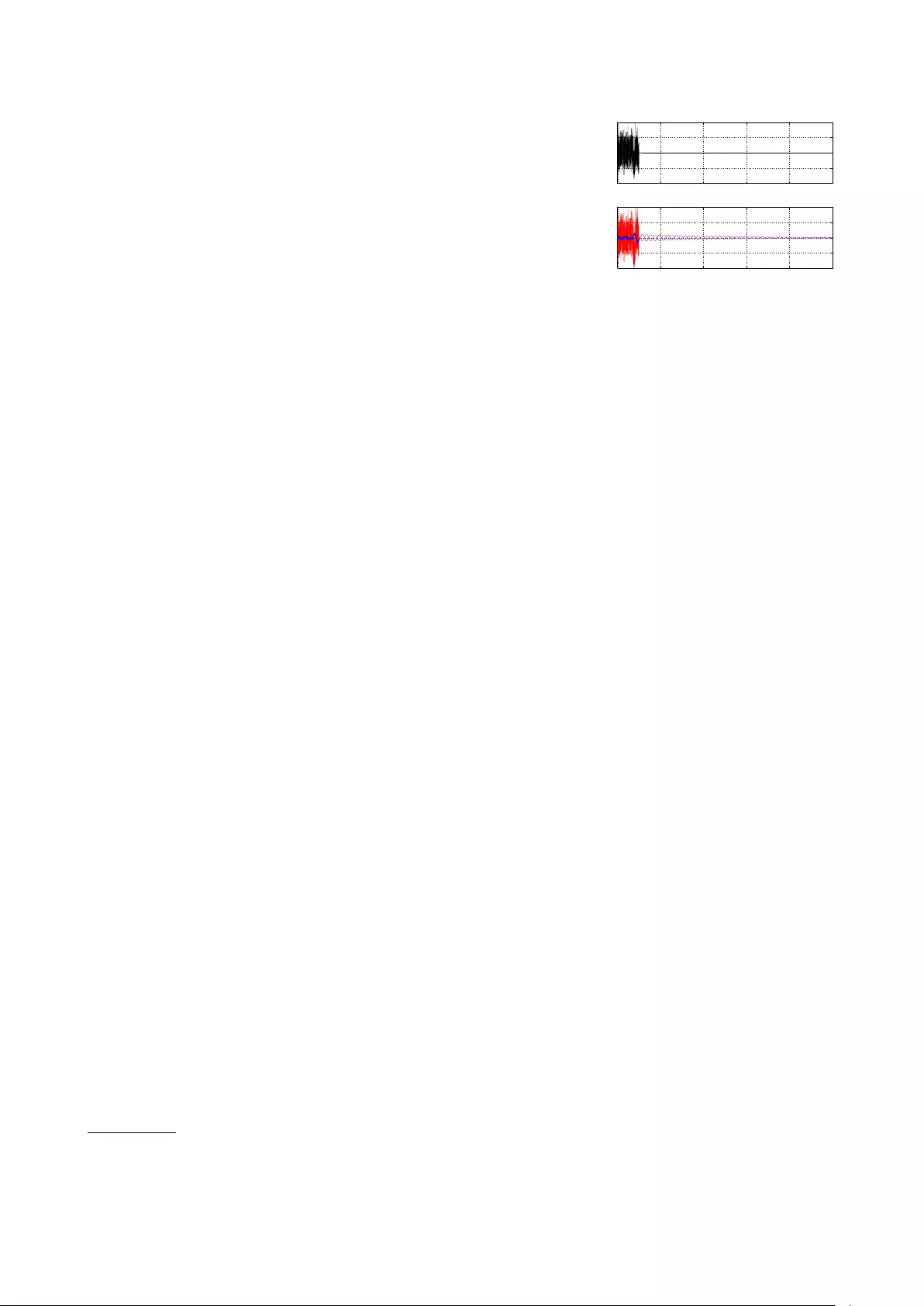
measurement with a microphone is not
necessarily representative of what
you’ll hear. This is because a
microphone does not have two ears.
Also, the direction the reflection comes
from will change how you perceive it. A
sidewall reflection sounds different
from a floor reflection. This is because
you have two ears – one on each side
of your head. Your brain uses the
sidewall reflections (or, more precisely,
how they relate to the direct sound) to
determine, in part, how far away a
sound source is. Also, since, in the
case of sidewall reflections, your two
ears get two different delay times on
the reflection (usually), you get two
different comb-filter patterns, where
the peaks in one ear can be used to fill
in the notches in the other ear and vice
versa. When the reflection comes from
the floor or ceiling, your two ears get
the same artefacts (since your two
ears are the same distance to the floor,
probably). Consequently, it’s easily
noticeable (and it’s been proven using
science!) that a floor or ceiling
reflection has a bigger timbral effect
on a loudspeaker than a lateral (or
sideways) reflection.
15.2 Room Modes
Room modes are a completely different
beast – although they exist because of
reflections. If you pluck a guitar string,
you make a deflection in the string that
moves outwards until it hits the ends of
the string. It then bounces back down
the string, bounces again, etc. etc. As
the wave bounces back and forth, it
settles in to a total result where it looks
like the string is just bouncing up and
down like a skipping rope. The longer
the string, the lower the note, because
it takes longer for the wave to bounce
back and forth on the string. You can
also lower the note by lowering the
tension of the string, since this will slow
down the speed of the wave moving
back and forth on it. The last way to
lower the note is to make the string
heavier (e.g. by making it thicker) –
since a heavier string is harder to
move, the wave moves slower on it.
The air in a pipe behaves exactly the
same way. If you “pluck” the air in the
middle of a pipe (say, by clapping our
hands, or coughing, or making any
noise at all) then the sound wave
travels along the pipe until it hits the
end. Whether the end of the pipe is
capped or not, the wave will bounce
back and travel back through the pipe
in the opposite direction from whence
it came.1As the wave bounces back
and forth off he two ends of the pipe, it
also settles down (just like the guitar
string) into something called a
“standing wave”. This is the pipe’s
equivalent of the skipping rope
behaviour in the string. The result is
that the pipe will “resonate” or ring at
a note. The longer the pipe, the lower
the note because the speed of the
sound wave moving in air in the pipe
stays the same, but the longer the
pipe, the longer it takes for the wave to
bounce back and forth. This is basically
how all woodwind instruments work.
What’s interesting is that, in terms of
resonance, a room is basically a big
pipe. If you “pluck” the air in the room
(say, by making sound with a
loudspeaker) the sound wave will move
down the room, bounce off the wall, go
back through the room, bounce of the
opposite wall, etc. etc. (Of course,
other things are happening, but we’ll
ignore those.) This effect is most
obvious on a graph by putting some
sound in a room and stopping
suddenly. Instead of actually stopping,
you can see the room “ringing”
(exactly in the same way that a bell
rings when it’s been hit) at a frequency
that gradually decays as time goes by.
However, it’s important to remember
that this ringing is always happening –
even while the sound is playing. So, for
example, a kick drum “thump” comes
out of the speaker which “plucks” the
room mode and it rings, while the
music continues on.
Time (sec)
Figure 15.8: The concept of the effect
of a room mode and Active Room Com-
pensation. See the associated text for
an explanation.
Figure 15.8 shows the concept of the
effect of a room mode and how it’s
dealt with by Active Room
Compensation. The sound coming out
of the loudspeaker is shown on the top
plot, in black. The response of the
loudspeaker and a single room mode is
shown below, in red. You can see there
that the room mode keeps “ringing” at
one frequency after the sound from the
loudspeaker stops.
There are two audible effects of this.
The first is that, if your music contains
the frequency that the room wants to
resonate at, then that note will sound
louder. When you hear people talk of
“uneven bass” or a “one-note-bass”
effect, one of the first suspects to
blame is a prominent room mode.
The second is that, since the mode is
ringing along with the music, the
overall effect will be muddiness. This is
particularly true when one bass note
causes the room mode to start ringing,
and this continues when the next bass
note is playing. For example, if your
room room rings on a C#, and the bass
plays a C# followed by a D – then the
room will continue to at C#, conflicting
with the D and resulting in “mud”. This
is also true if the kick drum triggers the
room mode, so you have a kick drum
“plucking” the room ringing on a C# all
through the track. If the tune is in the
key of F, then this will not be pretty.2
In order for the loudspeaker to
compensate for the effect of the room
mode, it has to not only produce the
1Whether the pipe is closed (capped) or open only determines the characteristic of the reflection – there will be a reflection either way.
2Do a search for “tritone” or “diabolus in musica”.
47
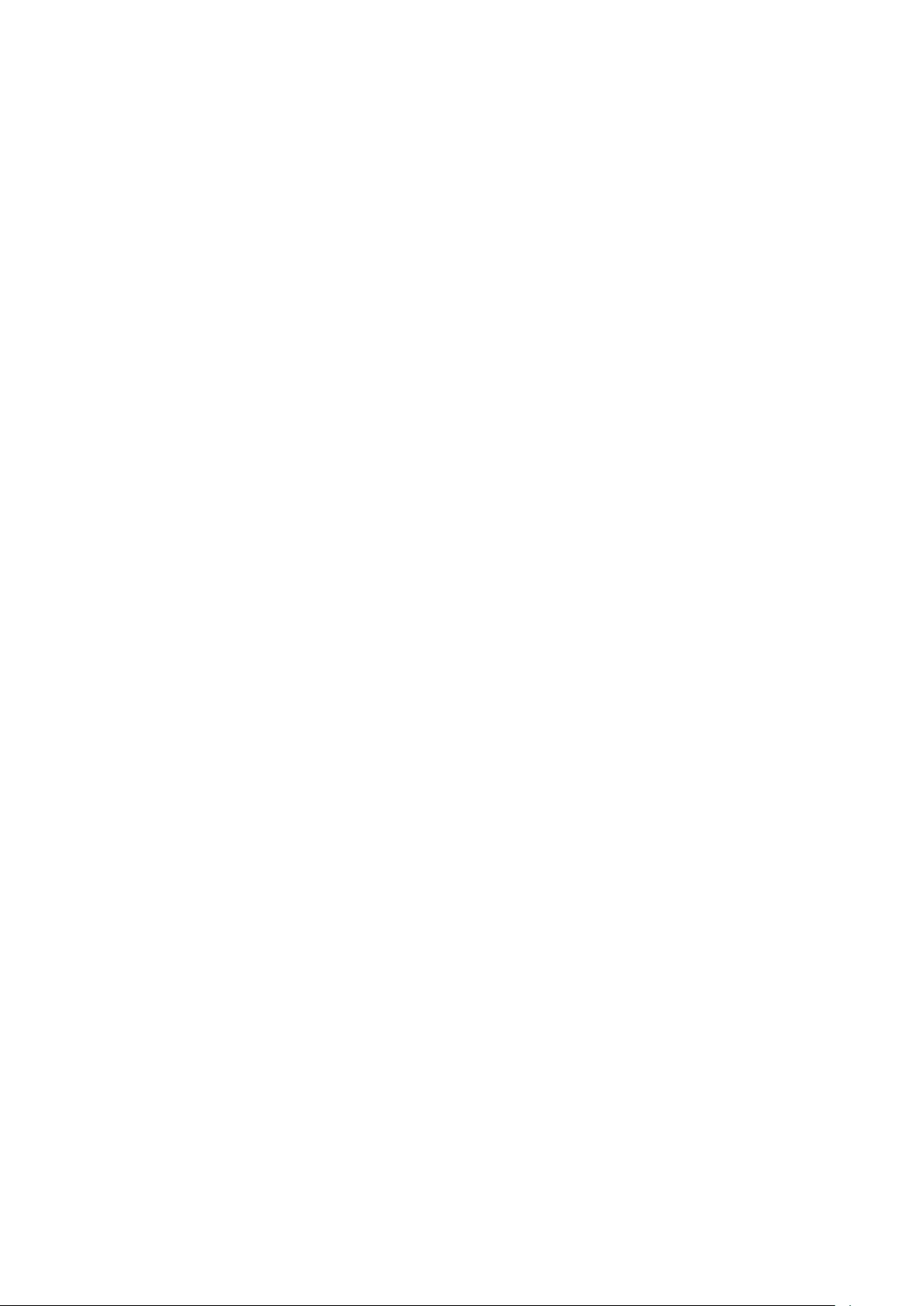
signal it should (shown in black) but it
must also produce a signal that
counter-acts the ringing in the room
mode. This is shown in the lower plot
in blue. As can be seen there (most
easily in the ringing after the signal
has stopped), the loudspeaker’s
compensation signal (the blue curve) is
the mirror image of the room’s
“misbehaviour” (in red). If you add
these two curves together, the result is
that they cancel each other out, and
the result is the black curve.
If you would like to calculate a
prediction of where you’ll have a
problem with a room mode, you can
use the following equation:
•metric version:
frequency = 172 / (length in m)
•imperial version:
frequency = 558 / (length in feet)
This calculation will produce the
fundamental frequency of the room
mode in Hz for the dimension of the
room represented by “length”. Your
most audible modal problems will be at
the frequencies calculated using either
of the equations above, and multiples
of them (e.g. 2 times the result, 3
times the result, and so on).
So, for example, if your room is 5 m
wide, your worst-case modes (for the
room’s width) will be at 172 / 5 = 34.4
Hz, as well as 68.8 Hz, 103.2 Hz and so
on. Remember that these are just
predictions – but they’ll come pretty
close. You should also remember that
this assumes that you have completely
immovable walls and no absorption – if
this is not true, then the severity of the
actual problem will vary accordingly.
Sadly, there is not much you can do
about room modes. There are ways to
manage them, including, but not
exclusive to the following strategies:
•make sure that the three
dimensions of your listening
room are not related to each
other with simple ratios
•put up membrane absorbers or
slot absorbers that are tuned to
the modal frequencies
•place your loudspeaker in a node
– a location in a room where it
does not couple to a problematic
mode (however, note that one
mode’s node is another mode’s
antinode)
•sit in a node – a location in a
room where you do not couple to
a problematic mode (see warning
above)
•use room correction DSP software
such as ARC in the BeoLab 50
15.3 Reverberation
Reverberation is what you hear when
you clap your hands in a big cathedral.
It’s the collection of a lot of reflections
bouncing from everywhere as you go
through time. When you first clap your
hands, you get a couple of reflections
that come in separated enough in time
that they get their own label – “early
reflections”. After that, there are so
many reflections coming from so many
directions, and so densely packed
together in time, that we can’t
separate them, so we just call them
“reverberation” or “reverb” (although
you’ll often hear people call it “echo”
which is the wrong word to use for this.
Reverb is what you get when you have
a lot of reflective surfaces in your room
– but since it’s so irregular in time and
space, it just makes a wash of sound
rather than a weird comb-filter effect
like we saw with a single reflection. So,
although it makes things “cloudy” – it’s
more like having a fog on your glasses
instead of a scratch, or a soft-focus
effect on a kitschy photograph of a
field of flowers.
15.4 Solutions
As we’ve seen, if your listening room is
normal, you have at least these three
basic acoustic problems to deal with.
Each problem has a different solution...
The first solution has already been
started for you. As is explained in the
section on Sound Design, the final
tuning of every Bang & Olufsen
loudspeaker (including the BeoLab 50)
is voiced in at least four rooms with
very different acoustical behaviours
ranging from a very “dead” living room
with lots of absorptive and diffusive
surfaces to a larger and very “live”
space with a minimalistic decorating,
and large flat surfaces. Once we have
a single sound design that is based on
the common elements those rooms,
we test the loudspeakers in more
rooms to ensure that they’ll behave
well under all conditions.
The second solution is BeoLab 50’s
Active Room Compensation which will
correct the effects of boundaries
(walls) and room modes on the timbre
of the loudspeaker at the listening
position(s). Using measurements of the
characteristics of the loudspeaker at
the listening positions, the ARC
algorithm then creates a filter that is
used to “undo” these effects. For
example, if the loudspeaker is close to
a wall (which will generally result in a
boosted bass) then the filter will
reduce the bass symmetrically.
Similarly, ringing caused by room
modes will be actively cancelled by
both BeoLab 50’s. That way, the loss in
the filter and the gain due to the room
will cancel each other.
The third solution is unique to the
BeoLab 50 – Beam Width Control. This
allows you to customise the relative
levels of the direct sound and the
reflected sound at the listening
position. The result of this is that, even
if you have acoustically reflective side
walls, the BeoLab 50 can still deliver
an accurate and precise representation
of the spatial presentation of your
stereo recordings.
15.5 Conclusions
Of course, this section does not cover
everything there is to know about room
acoustics. And, of course, you can’t
expect a loudspeaker to sound exactly
the same in every room. If that were
true, there would be no such thing as a
48
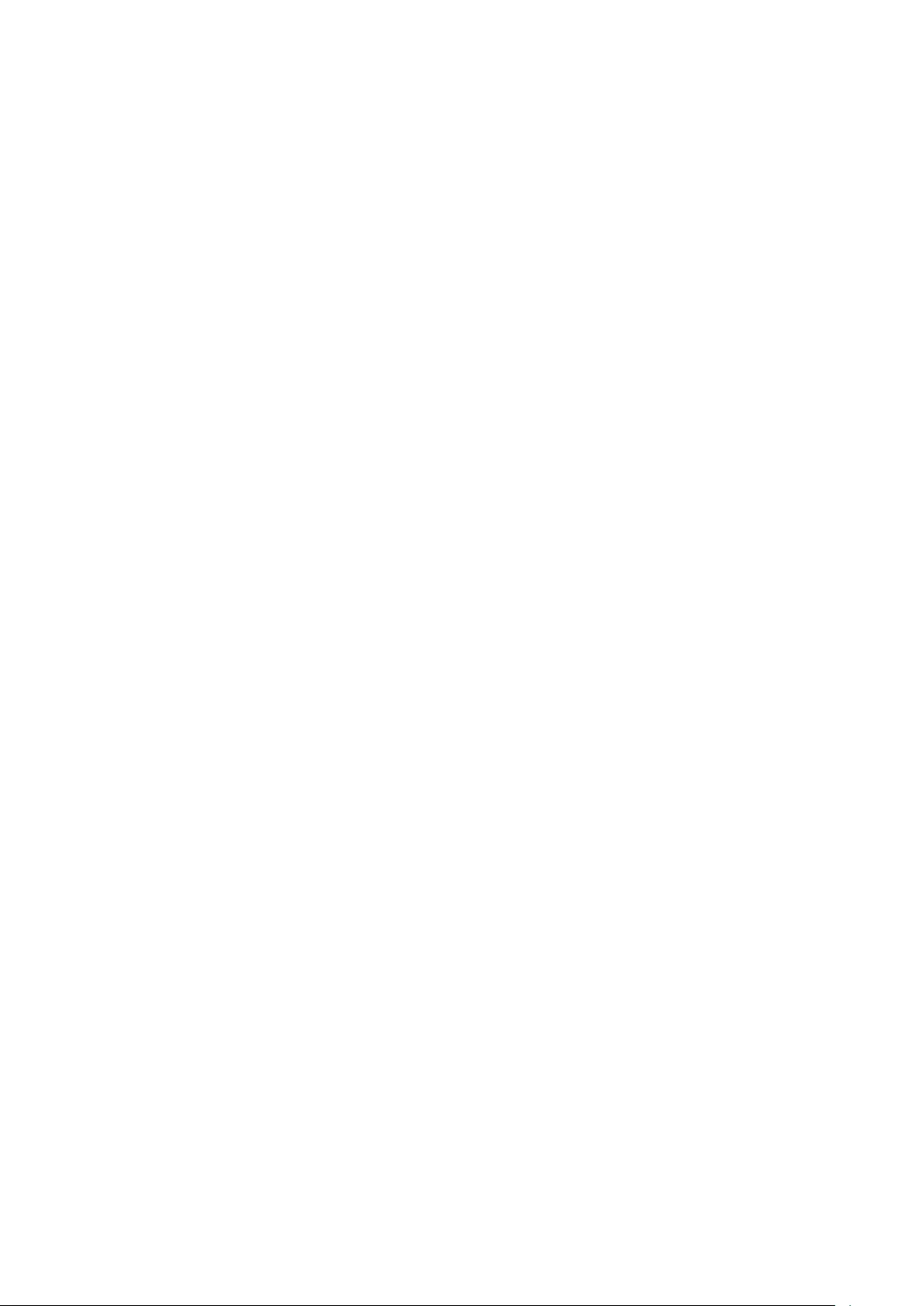
“good” concert hall. A room’s
acoustical behaviour affects the sound
of all sound sources in the room. On
the other hand, humans also have an
amazing ability to adapt – in other
words you “get used to” the
characteristics of your listening room.
However, there is no debate that, due
to many issues (the first two that come
to mind are frequency range and
directivity) two different loudspeakers
will behave differently from each other
in two different rooms. In other words,
if you listen to loudspeaker “A” and
loudspeaker “B” in a showroom of a
shop, you might prefer loudspeaker “A”
– but if you took them home, you might
prefer loudspeaker “B”. This would not
be surprising, since what you hear is
not only the loudspeaker but the
loudspeaker “filtered” by the listening
room. This is exactly why, even with
automated room compensation
algorithms, some fine tuning may be
necessary to achieve a sound that best
suits your room and your tastes.
49
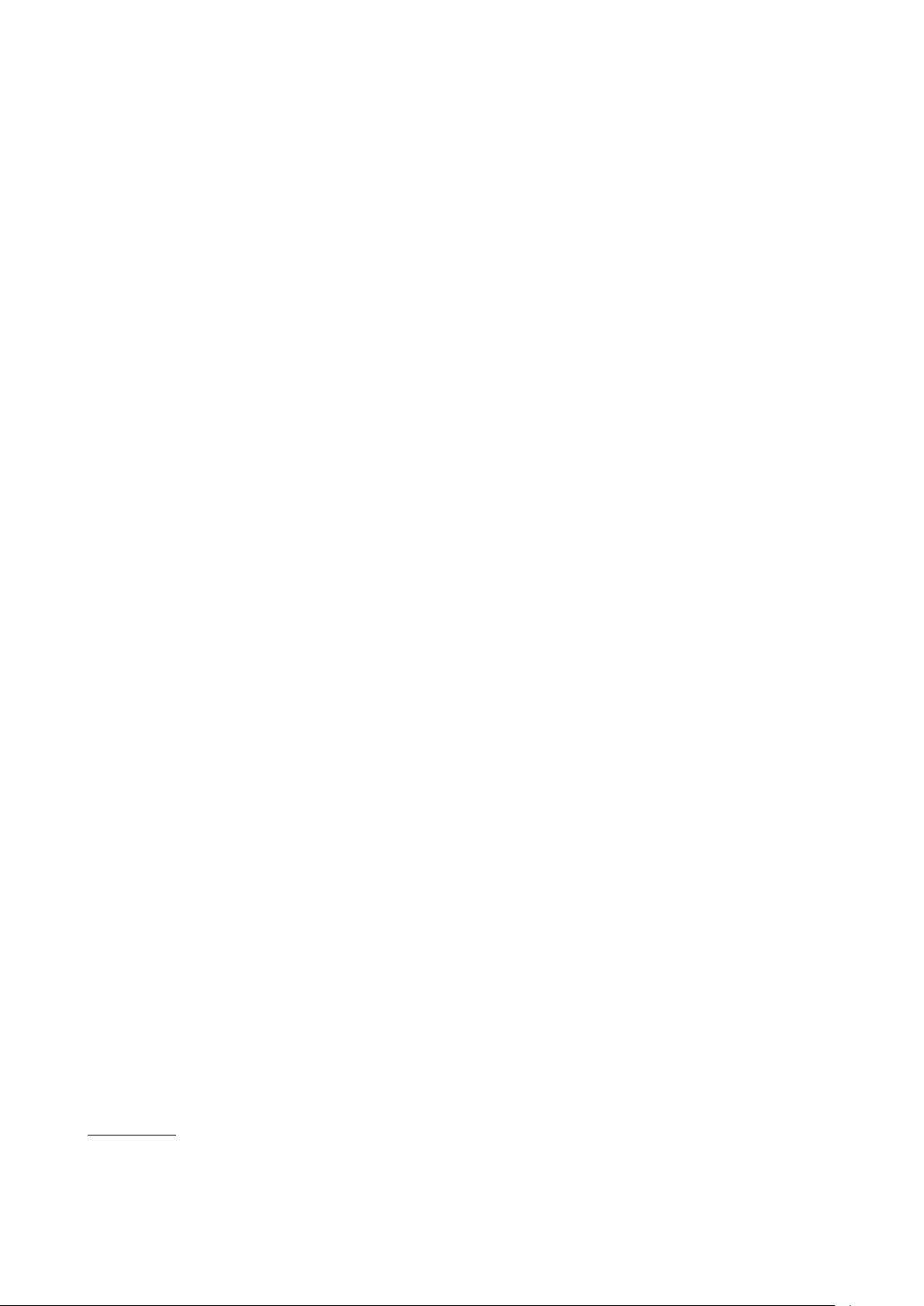
Appendix 4: Loudspeaker Directivity and Distance Perception in Stereo Imaging
16.1 Distance Perception in
Real Life
Go to the middle of a snow-covered
frozen lake with a loudspeaker and a
friend. Sit there and close your eyes
and get your friend to place the
loudspeaker some distance from you.
Keep your eyes closed, play some
sounds out of the loudspeaker and try
to estimate how far away it is. You will
be wrong (unless you’re VERY lucky).
Why? It’s because, in real life with real
sources in real spaces, distance
information (in other words, the
information that tells you how far away
a sound source is) comes mainly from
the relationship between the direct
sound and the early reflections from
walls in your listening room. If you
don’t have any early reflections, then
you don’t have any distance
information. Add the early reflections
and you can very easily tell how far
away it is.1
16.2 Distance Perception in a
Stereo Recording
Recording engineers have a basic trick
for controlling the apparent distance to
a sound source in a stereo recording
using the so-called “dry-to-wet” ratio
— in other words, the relative levels of
the direct sound and the reverberation.
To be honest, this is a bit of an
over-simplification, but it’s at the level
of knowledge one would typically have
if one were just starting out recording a
budding rock band in a garage.
Many classical recordings are made
with a pair of microphones. An
instrument that is on the left side of
the pair will produce a sound that is
slightly louder or slightly earlier in the
left microphone than in the right
microphone. This means that, when
you sit in the sweet spot and listen to
the stereo recording, you will hear that
source on the left side of the stereo
image. This effect is true not only for
the direct sound of the instruments
arriving at the microphone pair, but
also for the acoustic reflections off the
various surfaces in the recording
space. So, if the recording engineer
has been paying attention, the
distance information (the relationship
between the direct sound and the
reflections) has been captured in the
recording. This means that when you
listen to the recording, you not only
can tell where the instruments are
from left to right, but also their relative
distances.
16.3 Combining the Two
So, we know that early reflections tell
your brain how far away the sound
source is. Now think to a loudspeaker
in a listening room:
Case 1: If you have a listening room
that has no sidewalls, then there are
no early reflections, and, regardless of
how far away the loudspeakers are, a
sound source in the recording without
early reflections (e.g. a close-mic’ed
vocal) will sound closer to you than the
loudspeakers.
Case 2: If you have a listening room
with early reflections, and the
loudspeakers are less directional such
as BeoLab 50’s with their Beam Width
set to Wide or Omni, then the early
reflections from the side walls tell you
how far away the loudspeakers are.
Therefore, the close-mic’ed vocal track
from Case 1 cannot sound any closer
than the loudspeakers – your brain is
too smart to be told otherwise.
Case 3: If you have a listening room
with sidewalls and therefore early
reflections, but the loudspeakers are
directional such that there is no energy
being delivered to the side walls, then
the result is the same as in Case 1.
This time there are no early reflections
because of loudspeaker directivity
instead of wall absorption, but the
effect at the listening position is the
same. This is the case with BeoLab 50
when its Beam Width is set to Narrow.
The conclusion is that, in order to get
an accurate and precise representation
of the spatial properties in a stereo
recording, you should try to minimise
the levels of the early reflections from
the sidewalls in your listening room.
However, this means that you are
optimising the sound for the sweet
spot – on-axis to both loudspeakers.
When listening with friends, it may be
necessary to widen the loudspeakers’
Beam Widths.
1This has been proven in various listening tests. For example, go check out “Psychoacoustic Evaluation of Synthetic Impulse Responses” by Per Rubak & Lars G. Johansen as a
starting point.
50
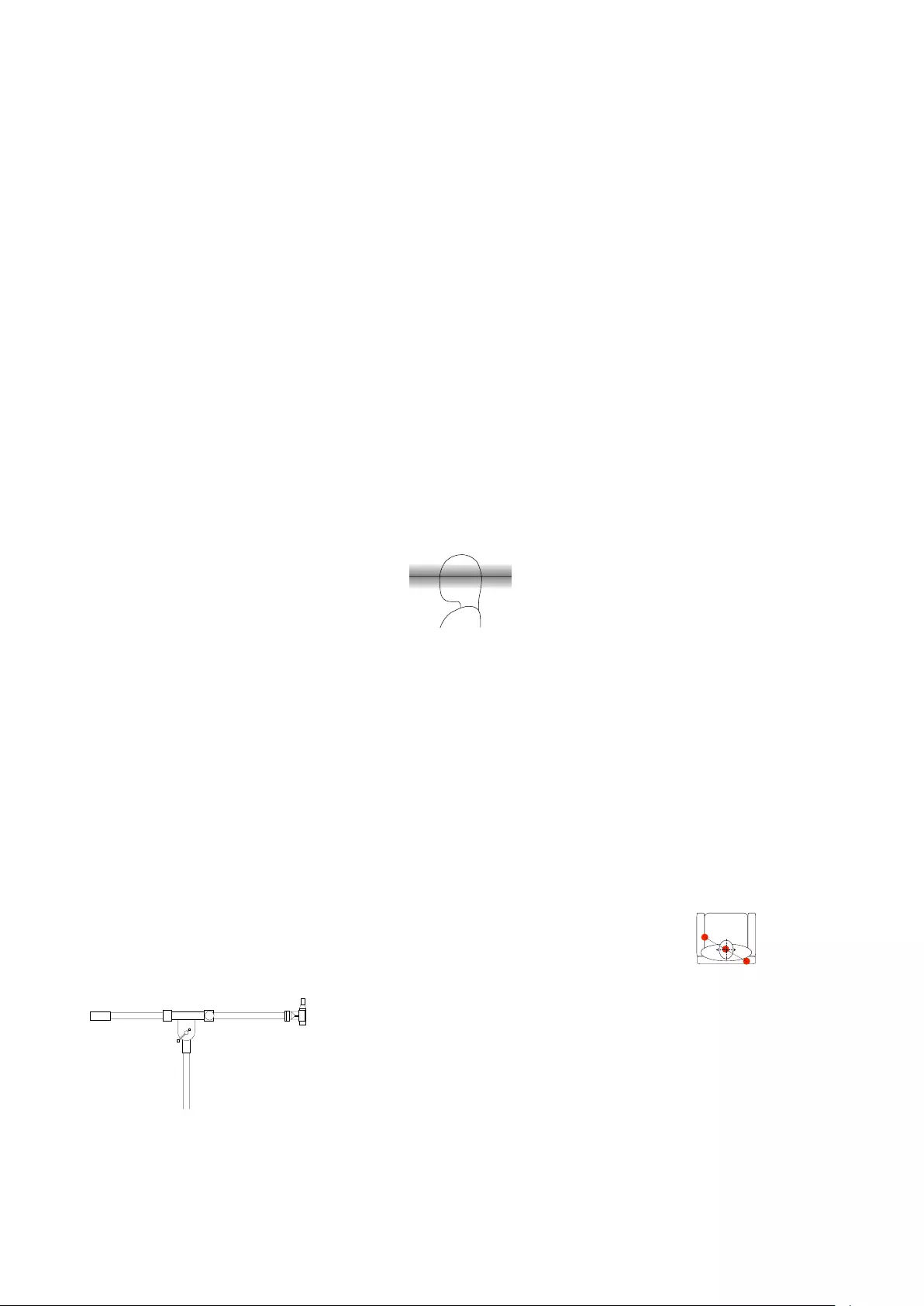
Appendix 5: Microphone placement strategy when creating ARC Zones
As is discussed in Active Room
Compensation, it is possible to create
settings for different ARC Zones (or
listening areas). This is done by
placing a microphone in three different
locations within the zone and
performing an ARC measurement at
each position. This section gives some
recommendations regarding where to
place the microphone for the
measurements.
17.1 General information
17.1.1 Background noise
It is important to ensure that
extraneous background noise is kept to
a minimum during the measurement
procedure. This does not only include
mid-range frequencies (e.g. speech)
but also low-frequency noise.
Therefore, for example, it is
recommended that air conditioning
systems be turned off, and the
measurements are performed during
low-traffic hours. This is because, in
some cases, the measurement may
interpret background noise as artefacts
of the room’s acoustical behaviour.
(For example, the process may result
in reduced bass in the loudspeakers if
a truck was idling outside the room
during the measurement procedure.)
17.1.2 Microphone
Orientation and Holder
The microphone should be securely
held (e.g. on a camera tripod or
microphone stand), pointing upwards
as is shown in Figure 17.1.
Figure 17.1: Recommended micro-
phone orientation.
It is not recommended that the
microphone be hand-held due to the
length of the measurement procedure
and the fact that the microphone
should not move during the
measurement. Extraneous noise
caused by holding the microphone may
also affect the measurement accuracy.
17.1.3 Height
If the ARC measurement is for only one
listener who never changes position
(e.g. never “slouches” in the listening
chair), then the height of the
microphone should be roughly the
same as the height of that person’s
ears, typically 100 – 120 cm above the
floor.
Figure 17.2: Recommended micro-
phone placement height for one listener
shown in gray.
If the ARC measurement zone is
intended for more than one listening
position, or for listeners of different
heights, then it may be beneficial to
change the vertical position of the
microphone for the three
measurements. For example, set the
centre microphone position at
ear-height, the front-left position
slightly lower (10-20 cm), and the
back-right position slightly higher
(10-20 cm). This will provide the
calculation with additional information
regarding the effects of room modes in
the vertical dimension that may
benefit listeners of different heights.
17.1.4 Doors and Windows
Doors and windows in the listening
room should have the same position
during the measurement as when the
room will be used for listening. So, if
you normally listen to music with the
doors closed, then they should also be
closed during the measurement
procedure. This is because opening a
door or a window can have a
significant effect on the acoustical
behaviour of a listening room.
If doors may be opened or closed for
different listening situations (e.g. patio
doors leading from the living room to
the outdoors) then two different ARC
Zones should be created separately for
the two different scenarios.
17.2 One listening position
If an ARC Zone consists of only one
listening position, it is recommended
that the three microphone positions
are:
•the location of the listener’s
head, as shown in Figure 17.3
•on each side of the listening
position (approximately 30 cm to
either side of the listening
position). One placement should
be slightly forward
(approximately 20 cm) and the
other should be slightly behind
(approximately 20 cm).
As mentioned above, the microphone
should be placed roughly at ear-height.
Figure 17.3: Recommended ARC micro-
phone placements (in red) for a single
listening position.
51
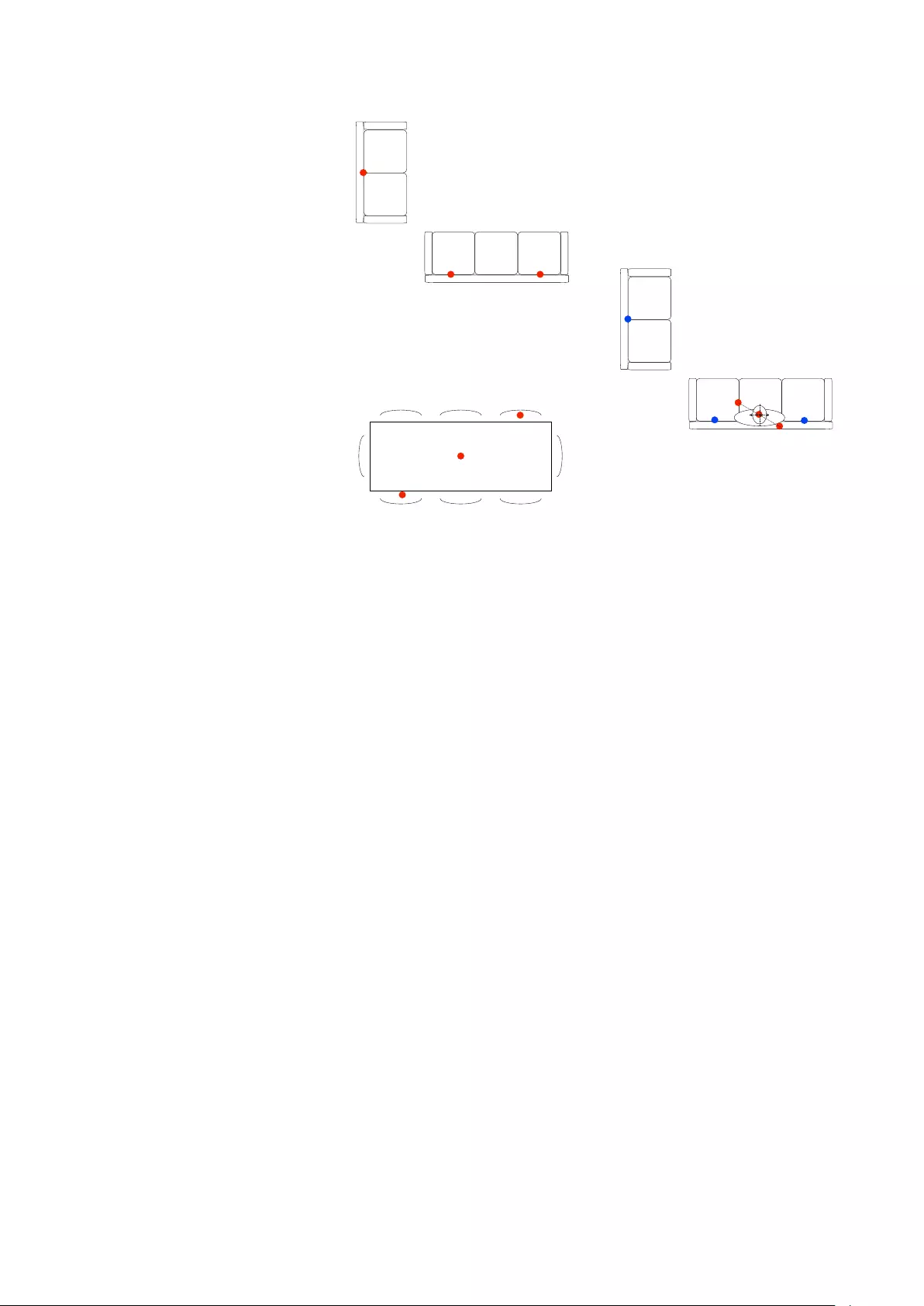
17.3 More than one listening
position
If an ARC Zone consists of more than
one listening position (e.g. a sofa) then
the measurement should be performed
once for each position. Figures 17.4
and 17.5 show a examples of zones
consisting of many possible listening
positions. The microphone should
placed at three positions (at
ear-height) distributed roughly evenly
throughout each zone to create the
ARC filter for each situation.
Figure 17.4: Recommended ARC micro-
phone placements (in red) for a multiple
listening positions.
Figure 17.5: Example ARC microphone
placements (in red) for a passive listen-
ing and background music situations at
a dining table.
Note that, in cases where there is
overlap between different ARC Zones,
the measurements can be combined
by combining ARC Profiles in the
BeoLab 50 interface instead of
duplicating measurements. An
example of this is shown in Figure 17.6.
Figure 17.6: An example of avoiding
duplicate microphone placements when
ARC Zones overlap. The “sweet spot”
zone is measured using the microphone
placements shown in red. The other lis-
tening positions on the sofas are mea-
sured for a second ARC Zone using
the microphone placements shown in
blue. These two are combined by se-
lecting both in the interface to com-
pletely cover the entire area.
52
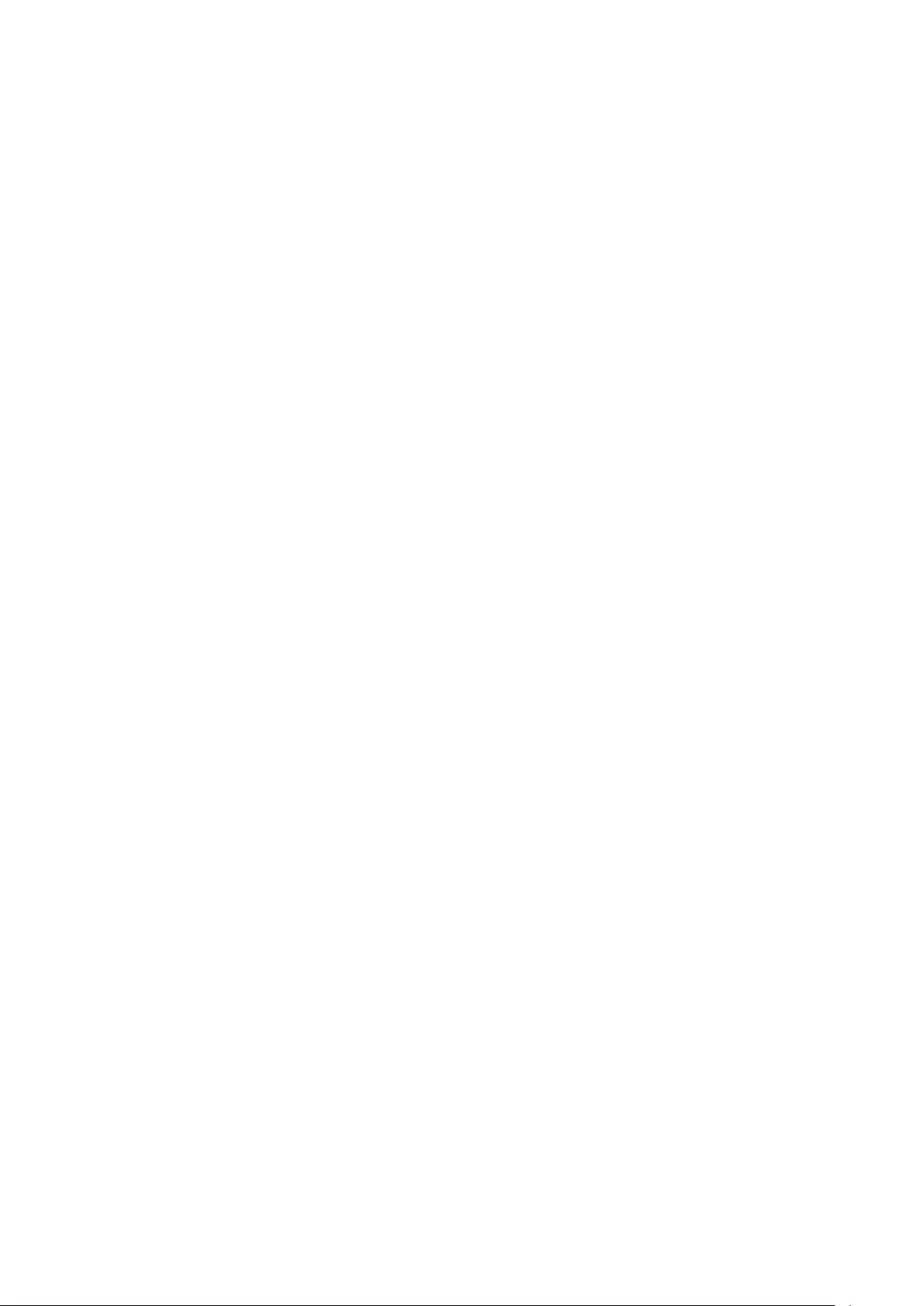
Appendix 6: ABL - Adaptive Bass Linearisation
18.1 A General Introduction
to ABL
Almost all loudspeakers made by Bang
& Olufsen include Adaptive Bass
Linearisation or ABL. This includes not
only our “stand alone” loudspeakers
(the BeoLab series) but also our
smaller “BeoSound” loudspeakers and
televisions. The only exceptions in the
current portfolio are our passive
loudspeakers, headphones, and the
BeoLab 5.
There is no one technical definition for
ABL, since it is in continual evolution –
in fact it may change from product to
product as we learn more and as
different products require different
algorithms. Speaking very broadly,
however, we could say that it reduces
the low frequency content sent to the
loudspeaker driver(s) (e.g. the woofer)
when the loudspeaker is asked to play
loudly – but even this is partially
inaccurate.
It is important to note that it is not the
case that this replaces a “loudness
function” which may (or may not) be
equalising for Equal Loudness Contours
(sometimes called “Fletcher-Munson
Curves”). However, since (generally)
the bass is pulled back when things get
loud, it is easy to assume this to be
true.
When we are doing the sound design
for a loudspeaker (which is based both
on measurements and listening), we
ensure that we are operating at a
listening level that is well within the
“linear” behaviour of the loudspeaker
and its components. (Typically, the
sound design is done at a standard
playback level where a -20 dB FS
full-band pink noise produces 70 dB (C)
at the listening position.)
This means that
•the drivers (usually the woofers)
aren’t being asked to move too
far (in and out)
•the amplifier is operating within
its limits
•the power supply is operating
within its limits, and
•nothing (not the power supply,
the amplifiers, nor the voice
coils) is getting so hot that the
loudspeaker’s behaviour is
altered.
This is what is meant by “linear” — it’s
fancy word for “predictable”. In
addition, it should be stated that if we
were listening to loudspeakers at high
levels daily, we would get increasingly
bad at our jobs due to hearing loss.
So, we do the tuning at a listening level
where we know things are behaving –
remember that we always do it at the
same calibrated level every time for
every loudspeaker so that we don’t
change sound design balance due to
shifts associated with equal loudness
contours. (If you tune a loudspeaker
when it’s playing loudly, you’ll wind up
with a loudspeaker with less bass than
if you tuned it quietly. This is because
you’re automatically compensating for
differences in your own hearing at
different listening levels.)
After that tuning is done, then we go
back to the measurements to see
where things will misbehave. For
example, in order to compensate for
the relatively small cabinet behind the
woofer(s) in the BeoSound 8 / BeoPlay
A8, we increase the amount of bass
that we send to the amplifiers for the
woofers as part of the sound design. If
we left that bass boost in the tuning
when you turn up the volume, the
loudspeaker would go up in smoke – or
at least sound very bad. This could be
because:
•the woofer is being pushed or
pulled beyond its limits, or
•because the amplifier clips or
•the power supply cannot supply
more current or
•something else.
So, after the tuning process is
complete, we put the loudspeaker in a
small torture chamber roughly the size
of a clothes closet, put on some dance
music (or modified synthetic test
signals) and turn up the volume. While
that’s playing, we’re continually
monitoring the signal that we’re
sending to the loudspeaker, the driver
excursion, the demands on the
electronics (e.g. the amp’s, DAC’s,
power supply, etc.) and the
temperature of various components in
the loudspeaker, along with a number
of other parameters.
Armed with that information, we are
able to “know” how those parameters
behave with respect to the
characteristics of the music that is
being played (e.g. how loud it is, in
various frequency bands, for how long,
in both the short term and the long
term). This means that, when you play
music on the loudspeaker, it “knows”:
•how hot it is at various locations
inside,
•what the velocity of the air in the
port is (if it has a port),
•the loudspeaker drivers’
excursions,
•amplifier demands,
•power supply demands,
•and so on. (The actual list varies
according to product – these are
just some typical examples...)
So, when some parameter gets close
to a maximum (e.g. the amplifier starts
to get too hot, or the woofer is nearing
maximum allowable excursion) then
something will be pulled back.
What is pulled back? It depends on the
product and the conditions at the time
you’re playing the music. It could be a
band of frequencies in the bass region,
it could be the level of the woofer. In a
worst-case-last-ditch situation, the
loudspeaker might even be required to
shut itself down to protect itself from
you (or the guests attending your
53
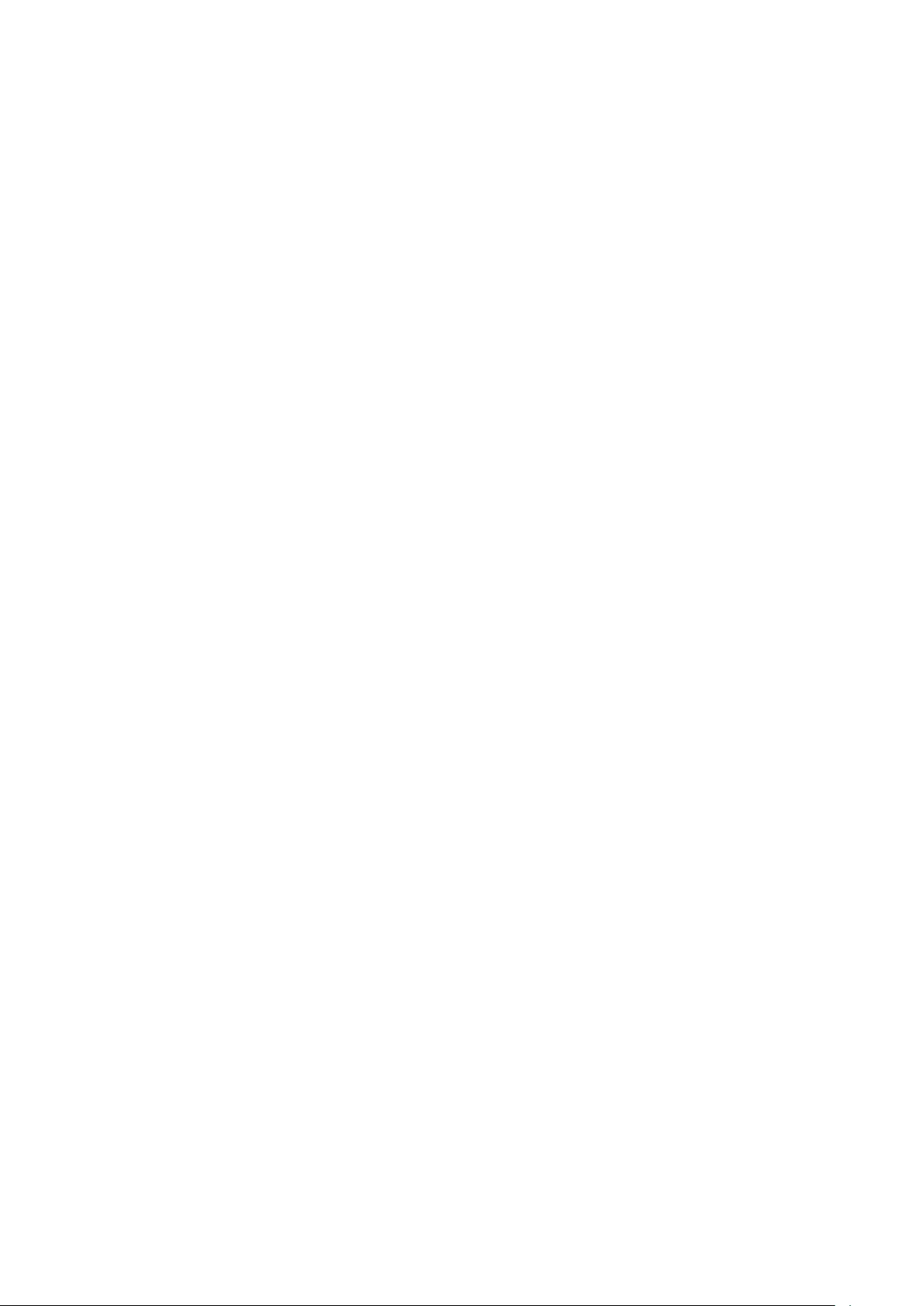
party). Of course, there is no
guarantee that you cannot destroy the
loudspeaker somehow – but we do our
best to build in enough protection to
cover as many conditions as we can.
How is it pulled back (i.e. how quickly
and by how much)? That also depends
on the product and some decisions we
made during the sound design process,
as well as what kind of
state-of-emergency your loudspeaker
is in (some people are very mean to
loudspeakers...).
Note that all this is done based on the
signals that the loudspeaker is being
asked to produce. So it doesn’t know
whether you’ve turned up the bass or
the volume – it just knows you’re
asking it to play this signal right now
and what the implications of that
demand are on the current conditions
(voice coil temperature, for example)
This is similar to the fact that the seat
belts in my car don’t know why the car
is stopping quickly – maybe it’s
because I hit the brakes, maybe it’s
because I hit a concrete wall – the seat
belts just lock up when they’re asked
to move too quickly. Your woofer’s
voice coil doesn’t know the difference
between Eminem and Stravinsky with a
bass boost – it just knows it’s hot and it
doesn’t want to get hotter.
18.2 ABL and BeoLab 50
In spite of BeoLab 50’s massive power
reserves and four capable woofers, it
still benefits from the inclusion of ABL
in its processing. This is due to the fact
that the BeoLab 50’s sound design
resulted in a frequency range that
extends to approximately 10 Hz.
Playing at high listening levels, such a
low frequency extension would result
in over-excursion of the woofers if ABL
were not included in the loudspeaker’s
processing. However, it should be said
that whereas a typical Bang & Olufsen
loudspeaker will have an ABL operating
at frequency bands from approximately
100 Hz and down, the BeoLab 50’s ABL
only operates below approximately 20
Hz.
54
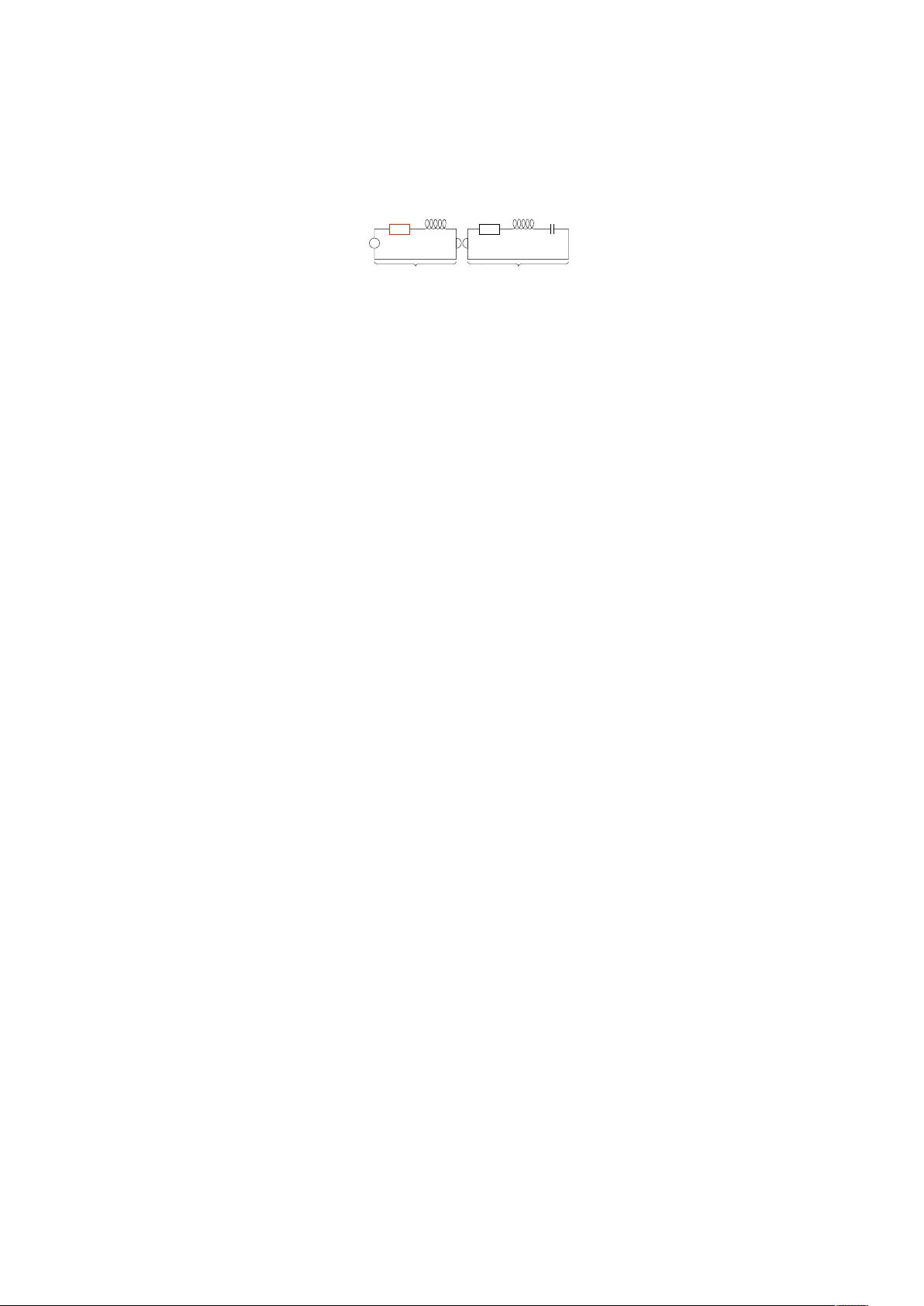
Appendix 7: Thermal Compression Compensation
19.1 Introduction
Take a woofer and put it in an
appropriately-sized cabinet, connect it
to an amplifier. Set the room
temperature to 20◦C. When everything
in the room is the same temperature,
measure the woofer’s on-axis
magnitude response.
Turn up the room temperature to
100◦C. When everything in the room is
the same temperature again, measure
the woofer’s magnitude response once
more.
You will notice that these two
measurements look very different – but
why?
When you read a magazine review of a
loudspeaker, it will include a
measurement of its “frequency
response” (more accurately called its
“magnitude response”) which shows
(ignoring many things) how loud
different frequencies are when they
come out of the loudspeaker assuming
that they all came in at the same level.
Unfortunately, this is only a small part
of the truth.
We can explain a loudspeaker driver’s
electromechanical characteristics by
breaking it down into different
components (both actual and
analogical). For example, the
suspension (which is comprised of the
surround and the spider) can be
thought of as a spring. The electrical
analogy for this is a capacitor. If you
take all of the moving parts in the
loudspeaker driver, they all add up to a
mass that has to be moved – the
electrical analogy for that mass is an
inductor (since an inductor has some
electrical “inertia”). Some of the
components are not an electrical
analogy – they are real electrical
components. For example, the voice
coil, since it’s a coil, acts as an
inductor. Since it is a thin bit of wire, it
also has some resistance to the flow of
electrical current through it, so it’s also
a resistor. A simple version of this
breakdown is shown in Figure 19.1.
Amplifier
Voice Coil
Resistance
Electrica Components Mechanical Components
Voice Coil
Inductance
Energy Losses in
Suspension
Inertia of Mass of
Moving Parts
Springiness of
Suspension
Figure 19.1: A simplified version of the
actual electrical and electrical analogies
of mechanical components in a loud-
speaker driver.
This shows the components of a
moving coil dynamic loudspeaker as a
very simplified “circuit” . If these
components don’t look familiar to you,
don’t worry, it’s not that important for
now. Some components in the circuit
are actual electrical things and others
are analogies – electrical
representations for a mechanical
component in the system.
If you know how each of these
components behaves, and you know
the correct values to put in for a given
loudspeaker, and you know how to do
the right math, then you can come
close to getting a prediction of the
response of the loudspeaker that
you’re modelling with the circuit.
However, if you just put in one value
for each component, then you’re
assuming that they never change – in
other words that you’re dealing with a
“linear” system.
The problem is that this assumption is
incorrect. For example, the voice coil
resistance – the amount that the wire
in the voice coil resists the flow of
current through it when the
loudspeaker driver is not moving –
changes with temperature. The hotter
the wire gets, the higher the resistance
goes. (This is a normal behaviour for
most resistors.) If the voice coil
resistance changes, then the whole
system behaves differently, since it
isn’t the only component in the circuit.
So, as we change the temperature of
the voice coil, the total response of the
loudspeaker changes.
Sadly, the temperature of the voice coil
isn’t only dependent on the room
temperature as it seemed to be in the
beginning of this discussion. As soon
as you start playing sound using the
loudspeaker, it starts heating up. The
louder the signal, the hotter it gets. So
as you play music, it heats and cools.
The speed with which it heats up and
cools down is dependent on its
“thermal time constant” – a big woofer
with a large voice coil and magnet will
take longer to heat up and cool down
(and therefore have a longer thermal
time constant) than a small tweeter.
This raises at least four questions:
•How much does the temperature
vary when I play music?
•How does the response of the
loudspeaker change with
temperature?
•How much does the response of
the loudspeaker change with
temperature?
•What can we do about it?
19.2 Voice coil temperature
A typical loudspeaker driver is, give or
take, about 1% efficient. This means
that approximately 1% of the power
you push into the loudspeaker from the
amplifier is converted into sound. The
remaining 99% is lost as heat – almost
all of it at the voice coil of the
loudspeaker. So, the louder your
music, the hotter your voice coil gets.
Of course, if you have a way of cooling
it (for example, by using other parts of
the loudspeaker as a radiator to your
listening room) then it won’t get as
hot, and it will cool down faster.
For example, play pop music that has
been mastered at a high level and play
it at maximum volume on a BeoLab 50
whilst monitoring the temperature of
the voice coils. What you’ll see if you
do this is something like the Figure
19.2.
55
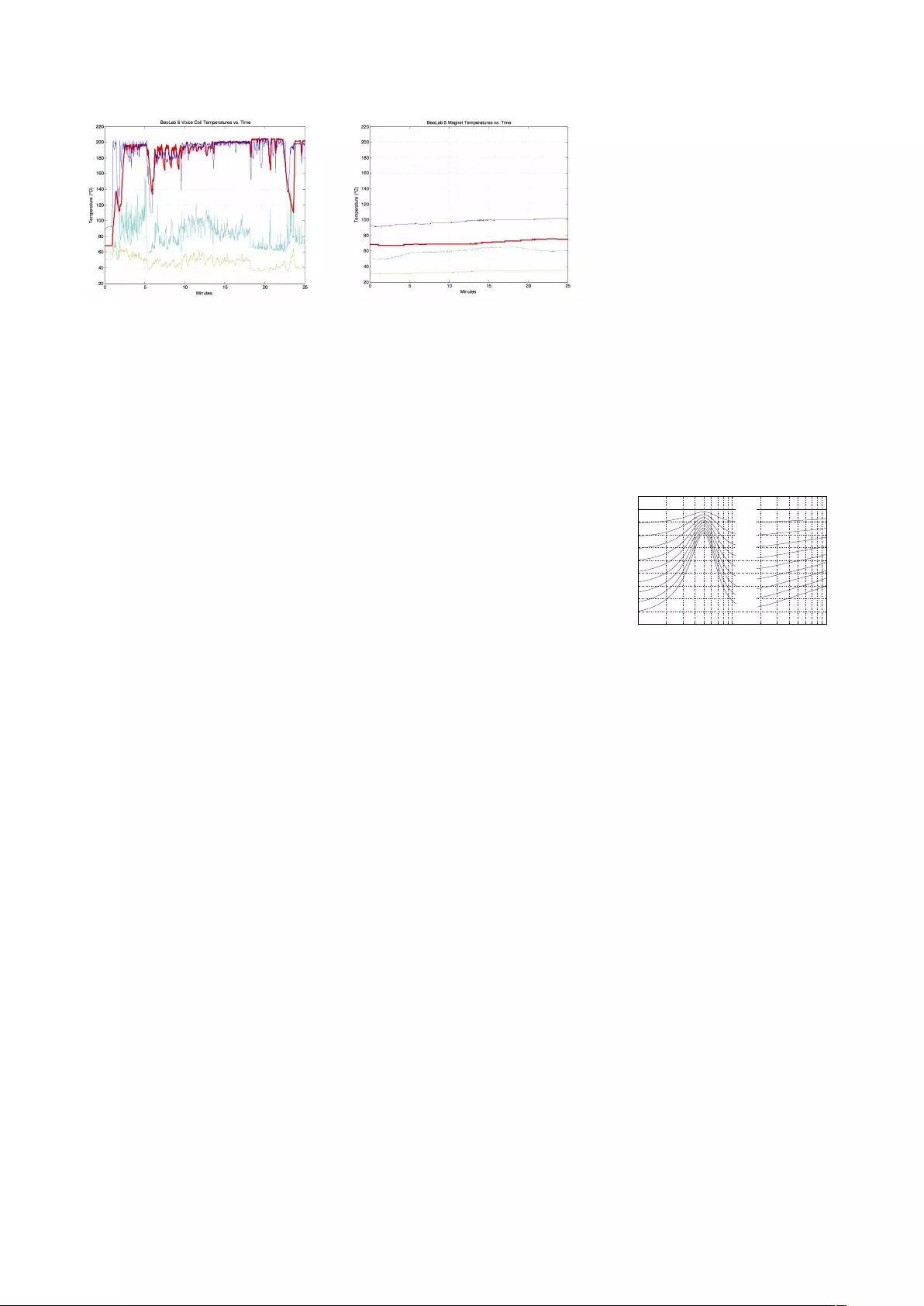
Figure 19.2: The temperatures (in ◦C)
of the voice coils of the drivers in a Be-
oLab 5 as a result of playing pop music
at full volume. The X-axis is the time in
minutes.
As you can see in Figure 19.2, while
playing music, the woofer varied from
a maximum temperature of about
200◦C down to about 110◦C.
This means that the worst-case
variation in temperature of the woofer
was about 90◦C whilst playing music,
and peaked at about 180◦C above
room temperature (which we’ll assume
is 20◦C).
Unfortunately, this temperature cannot
be measured directly, since we cannot
put thermal sensors directly on the
drivers’ voice coils. Instead, we
measure the temperature of the
loudspeaker driver magnets, and use
that real-time data input in addition to
the signal that we’re sending to the
drivers to calculate the temperatures
of the voice coils based on thermal
models of each of them. As you can
see in Figure 19.3, the magnet
temperature reacts much more slowly.
These measurements were taken at
exactly the same time as the ones
shown in Figure 19.2.
Figure 19.3: The temperatures of the
magnets of the loudspeaker drivers in a
BeoLab 5 as a result of playing pop mu-
sic at full volume. The X-axis is the time
in minutes.
19.3 Loudspeaker response
changes
So, now the question is “what does this
change in temperature do to the
response of the loudspeaker driver?”.
As I mentioned above, the thing that
changes most in the model shown in
Figure 19.1 is the loudspeaker driver’s
voice coil resistance. For those of you
with a background in reading electrical
circuits, you may notice that the one
shown in Figure 19.1 has some reactive
components in it which will result in a
resonance at some frequency. For
those of you without a background in
reading electrical circuits, what this
means is that a loudspeaker driver (like
a woofer) has some frequency at which
it “wants” to ring – if you thump it with
your thumb, that’s the note that you
will hear ringing – a little like a bell with
a low pitch.
As the voice coil resistance goes up, its
resistance increases, and we generally
lose sensitivity (i.e. level or loudness)
from the woofer. In other words, the
hotter it gets, the quieter it gets.
However, this only happens at the
frequencies where the resistor is not
“overridden” by another component –
say the mechanical resonance of the
woofer or the inductance of the voice
coil.
The total result is shown for various
temperature differences in Figure 19.4.
Notice that these plots show the
change in magnitude response of the
driver with changes in temperature.
So, the curve at the top is the change
in the woofer’s magnitude response
(which is 0 dB at all frequencies – in
other words no change) when the
loudspeaker is playing at the same
temperature at which it was measured
(let’s say, 20◦C or room temperature).
As the temperature of the voice coil
increases above that temperature, you
can see that you lose output in two
frequency bands on either side of a
“bump” in the response – that bump is
at the resonant frequency of the
loudspeaker driver.
So, the louder you play, the more low
end you lose, apart from a peak in the
response (which also rings in time) at
the resonant frequency of the driver.
10 1001,000
−4.5
−4
−3.5
−3
−2.5
−2
−1.5
−1
−0.5
0
0.5
Frequency (Hz)
Sensitivity (dB)
+ 0º C
+ 20º C
+ 40º C
+ 60º C
+ 80º C
+ 100º C
+ 120º C
+ 140º C
+ 160º C
+ 180º C
Figure 19.4: Sensitivity of BeoLab 50’s
front woofer vs. the change in temper-
ature of its voice coil.
19.4 The solution
Interestingly, everything I said above is
true for every moving coil loudspeaker.
So, if you’re the kind of person who
believes that the only “proper”
loudspeaker is one where you have
nothing but a loudspeaker driver (in a
cabinet of any kind, or not) and an
amplifier – and no active filtering, then
you’ll have to live with the kind of
unpredictable behaviour that you see
above. However, since a BeoLab 50
“knows” the temperature of the voice
coil of its loudspeaker drivers, and
since it has been programmed with the
curves like the one shown in Figure
19.4, we can actively linearise its
response, making it much more
predictable.
In essence all we need to do is to take
Figure 19.4, flip it upside down and
56
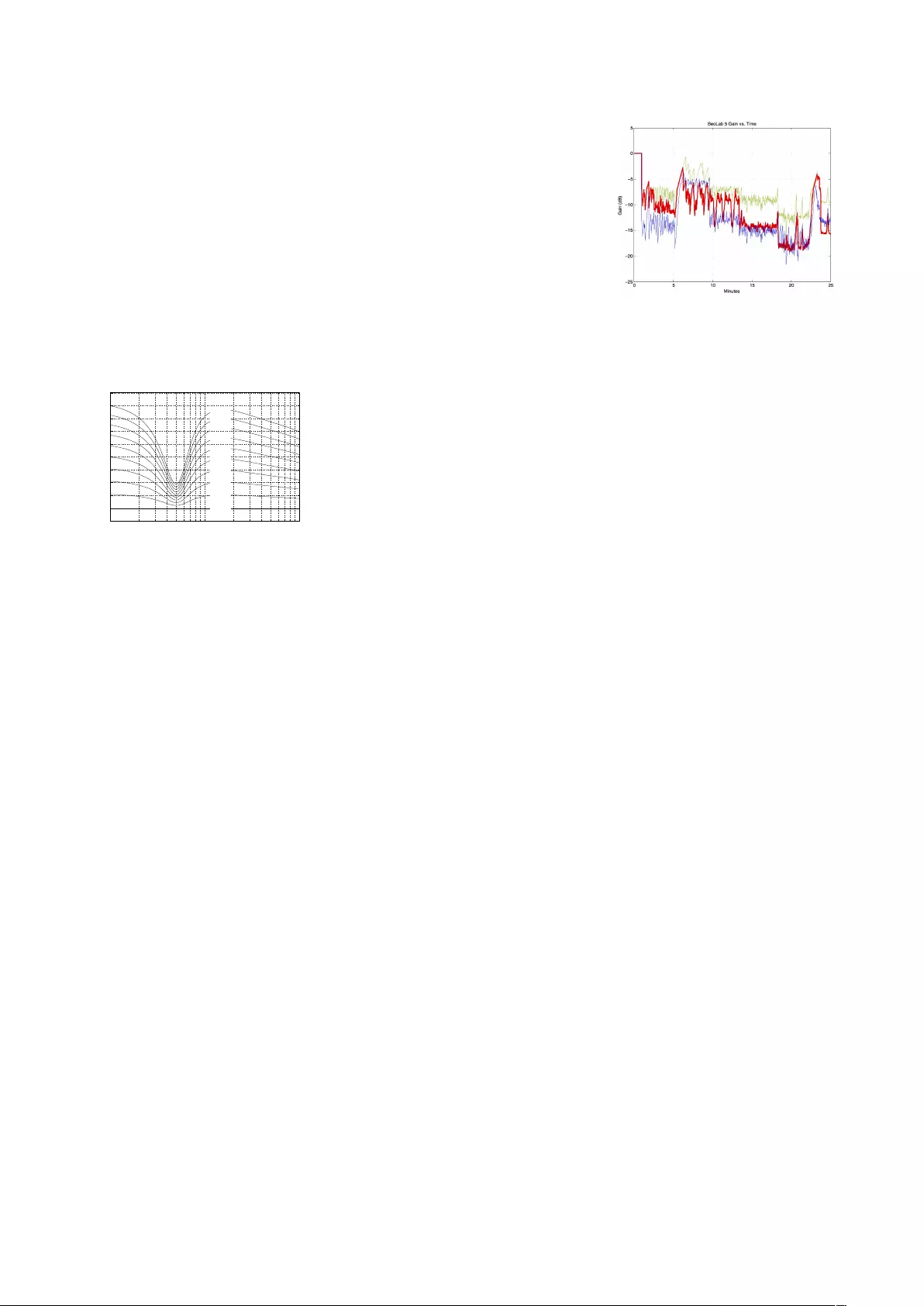
make a filter that “undoes” the effect
of temperature on the loudspeaker’s
response. For example, if the woofer’s
voice coil gets 160◦C above room
temperature (where we originally
measured it), it drops 3.2 dB at 20 Hz,
the BeoLab 50 knows this and adds 3.2
dB at 20 Hz. In order to do this, the
processing of the BeoLab 50 includes a
set of filters (one for each driver)
whose response varies in time with the
temperatures of the the drivers. The
temperature-dependent filters for the
front woofer are shown in Figure 19.5.
10 1001,000
−0.5
0
0.5
1
1.5
2
2.5
3
3.5
4
4.5
Frequency (Hz)
Gain (dB)
+ 0º C
+ 20º C
+ 40º C
+ 60º C
+ 80º C
+ 100º C
+ 120º C
+ 140º C
+ 160º C
+ 180º C
Figure 19.5: Magnitude responses of
the compensating filter for BeoLab 50’s
front woofer vs. the temperature of its
voice coil.
It’s important to note three things here.
•This can only be done because
we know how the response of the
woofer changes at different
temperatures (this behaviour
was found as part of the
development process).
•This can be done because the
loudspeaker “brain” (the DSP)
knows the temperature of the
voice coil in real time as you’re
playing music
•This particular filter shown in
Figure 19.5 should only be
applied to the appropriate
loudspeaker driver. The other
woofers and the other drivers
have different behaviours and
should be processed with their
own correction curves. In other
words, this filtering can only be
done because the BeoLab 50 is
an active loudspeaker with
independent filtering for each of
the 18 loudspeaker drivers.
19.5 Some extra information
You should be left with at least one
question. I said above that, as the
music gets loud, the woofer heats up,
so you lose output, so we add a filter
that compensates by putting more
signal into the driver. However, this
means that the problem is caused by
the signal being too loud, and the
result is that we make the signal
louder.
However, there is one more trick up
our sleeve. Appendix 6: ABL - Adaptive
Bass Linearisation describes BeoLab
50’s Thermal Protection algorithm. This
means that the DSP brain knows the
temperature of the drivers and, in a
worst-case situation, turns the levels
down to protect things from burning
up. So, if we go back to the example of
a BeoLab 50 playing at full volume,
let’s see what’s happening to the
signal levels.
Figure 19.6: The gains (in dB) applied to
the signals sent to the drivers in a Beo-
Lab 5 as a result of playing pop music
at full volume. The X-axis is the time in
minutes.
These curves in Figure 19.6 show the
gains applied to the front woofer in a
BeoLab 50 at the same time as the
measurements shown in Figures 19.2
and 19.3 were being made. In fact, if
you look carefully at Figure 19.2
around the 5 minute mark, you’ll see
that the temperature dropped – which
is why the gain in Figure 19.6 increases
(because it can!) in response.
Now, don’t panic. The BeoLab 50 isn’t
messing about with the gains of the
drivers all the time. Remember that
this example was done at full volume –
which, for a BeoLab 50 is extremely
loud. The gains shown in Figure 19.6
are a “last-ditch” effort of the
loudspeaker to protect itself from a
very mean customer (or the very mean
children of a customer who is away for
the weekend). This is the equivalent of
the airbags deploying in your car. You
can guess that, if the airbag is outside
the steering wheel something
significant has occurred.
Many thanks to Gert Munch for his help
in writing this section.
57
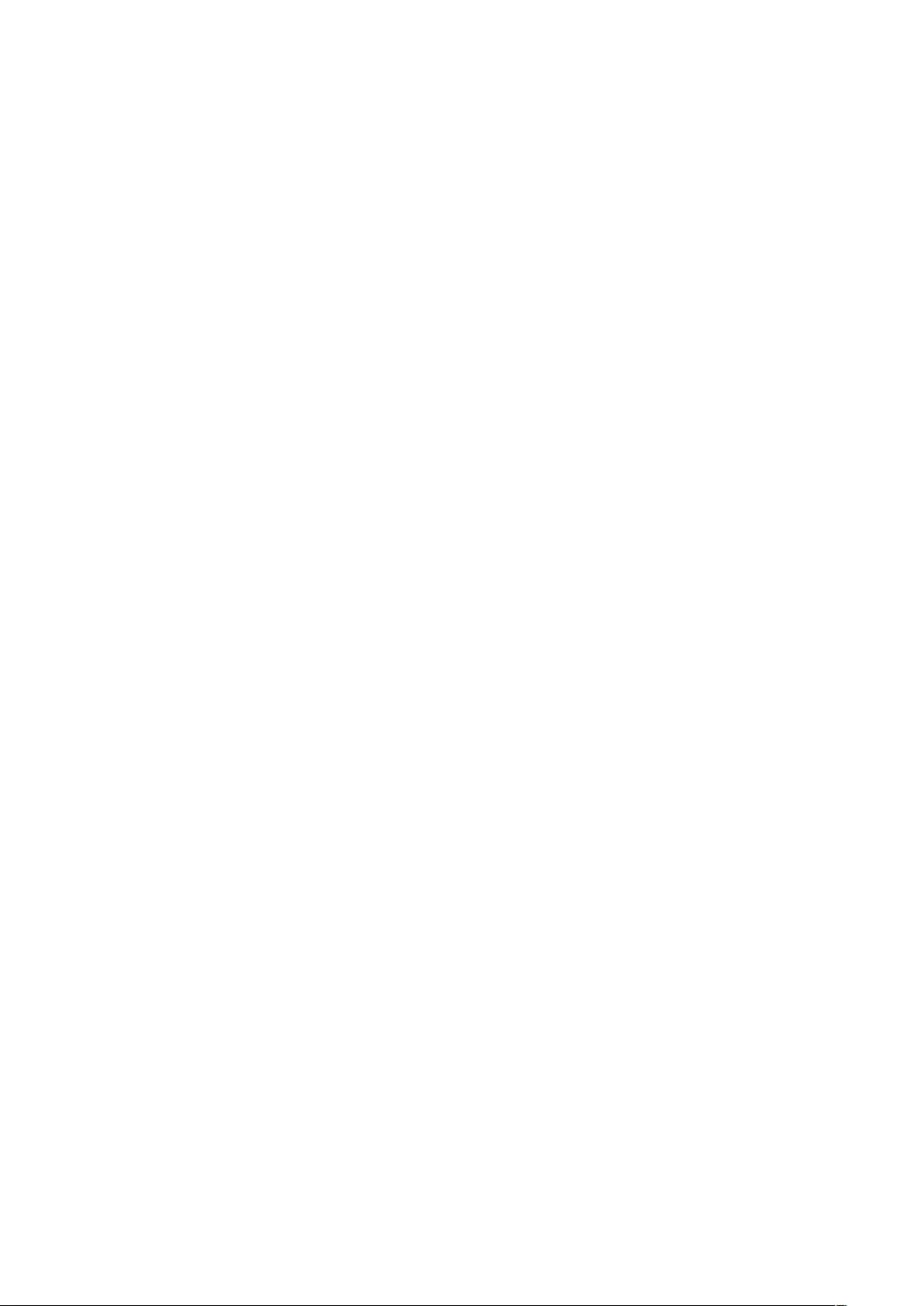
Appendix 8: Control of BeoLab 50 using the BeoRemote 1
20.1 Introduction
The BeoRemote 1 can be used instead
of the smartphone application to
control a number of parameters on the
BeoLab 50. The usage and setup of
these are described below
Note that, in order to control the
BeoLab 50’s with the BeoRemote 1,
two things must initially be configured.
Firstly, the infra-red receiver (also
known as an “IR Eye”) must be
correctly connected to the Master
BeoLab 50. Secondly, the BeoRemote
1 must be set to the “BeoSound”
product on its display. In addition, the
BeoLab 50 must be “paired” to the
BeoSound product (since there are
multiple BeoSound options).
20.1.1 Customising the
Product Name
It is possible to customise the product
name displayed on the BeoRemote 1
(for example, to read “BeoLab 50”
instead of “BeoSound”). This can be
done as follows:
1. Press LIST
2. Scroll down to SETTINGS and
press the round GO button
3. Scroll down to ADVANCED and
press GO
4. Select PRODUCTS and press GO
5. Select RENAME and press GO
6. Select the item you wish to
rename and press GO
7. “Type” in the new name for the
item
8. When you are done, scroll all the
way to the right of the list of
letters and select the CHECK
MARK to store.
20.2 Input Selection
It is possible to manually change the
input selection of audio sources using
the BeoRemote 1. In addition, the
display of the remote control can be
customised to reflect your particular
setup.
20.2.1 Source Selection
If you press the MUSIC button on the
remote, you will see a number of
sources listed there that are scrollable
using the UP/DOWN buttons, and
selectable using the GO button on the
remote control. Firstly, you will need to
customise this list as follows:
1. press LIST
2. Scroll down to SETTINGS and
press GO
3. Scroll down to MUSIC sources
and press GO
4. Select SHOW and press GO
5. Use the GO button to place a
check mark next to the following
items in the following list. (It may
also be helpful to remove the
check marks next to the items
that are not in the list.)
- NET RADIO
- MUSIC
- LINE IN
- CD
Now press the MUSIC button and you
should see these 4 items in a scrollable
list on the screen of the remote. These
4 are mapped to the inputs of the
BeoLab 50 as follows:
BL50 Input BR1 Display
Automatic Music
RCA Line-In
S/P-DIF CD
Optical NET Radio
So, for example, if the BeoLab 50 is in
Automatic mode and you playing
signals on both the S/P-DIF and the
RCA inputs simultaneously, you will
hear the S/P-DIF signal if it has a higher
selection priority. Press MUSIC and
select “Line-In” to switch manually to
the RCA input. Press MUSIC and select
CD to switch to the S/P-DIF input.
20.2.2 Customising the Input
Names
It is possible to re-name these inputs
on the BeoRemote 1’s display so that
you do not have to remember the
mapping. This can be done as follows:
1. Press LIST
2. Scroll down to SETTINGS and
press GO
3. Scroll down to MUSIC sources
and press GO
4. Select RENAME and press GO
5. Select the item you wish to
rename and press GO
6. “Type” in the new name for the
item
7. When you are done, scroll all the
way to the right of the list of
letters and select the CHECK
MARK to store.
Now, when you press the MUSIC
button, you will see the labels you
have entered instead of the default
ones listed above.
58
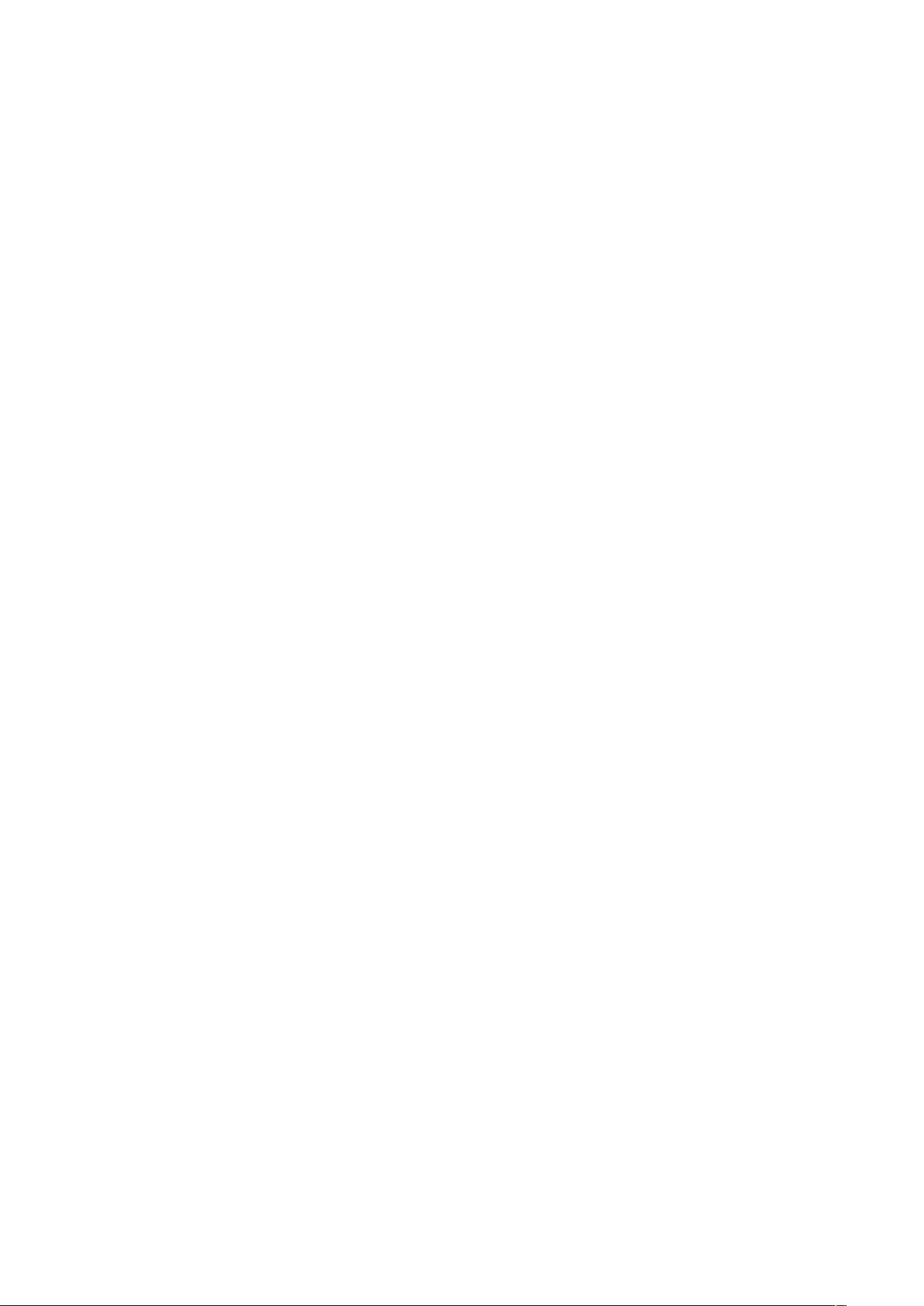
Index
ABL, 29,53
about, 27
active listening, 7
active room compensation, 8,15
Adaptive Bass Linearisation, 53
ADC, 32
Analogue Inputs, 32
analogue-to-digital converter, 32
ARC, 8,15,51
audio formats
DoP, 35,38
DSD, 35
DSD over PCM, 38
DXD, 35,38
automatic bass linearisation, 29
bass, 16
beam width
control, 7,12
narrow, 12
wide, 13
BeoRemote 1, 58
BeoVision television, connection to, 20
centre frequency, 43
cloning in production, 30
coaxial digital (S/P-DIF), 33
converter, digital to analogue, 33
DAC, 33
dB, 43
decibel, 43
digital inputs, 32
digital signal processor, 33
digital to analogue converter, 33
distance, 14
DSP, 33
early reflections, 45
echo, 37
edit mode, menu, 11
equaliser, parametric, 19,28
Fc, 43
features, 29
disabled, 37
filter
high-shelving, 20,42
low-shelving, 19,42
peaking, 20,43
phase response, 29
Q, 43
reciprocal peak/dip, 43
frequency tilt, 18
gain, 43
equaliser, 43
filter, 43
offset, 24
Hertz, 43
high-shelving filter, 20,42
Hz, 43
imaging, 7,12
impedance, input, 25
input
auto-detection, 24
detection threshold, 24
gain offset, 24
impedance, 25
maximum input voltage, 24
renaming, 23
time-out, 24
volume control, 25
input selection
manual, 23
priority, 23
input, labels, 23
latency, 17
level, 14
lip synch, 37
loudness, 18
low-shelving filter, 19,42
maximum input voltage, 24
menu map, 10
microphone placement, 51
minimum phase, 19
modes, room, 47
mute, 16
optical, 33
volume control via Power Link, 25
optical digital (optical), 33
parametric equaliser, 19,28
passive listening, 7
peaking filter, 20,43
phantom imaging, 12
Power Link, 32
preset, 11
automation, 20
creating, 11
edit parameters, 11
selecting, 11
production, 30
protection, thermal, 29
59
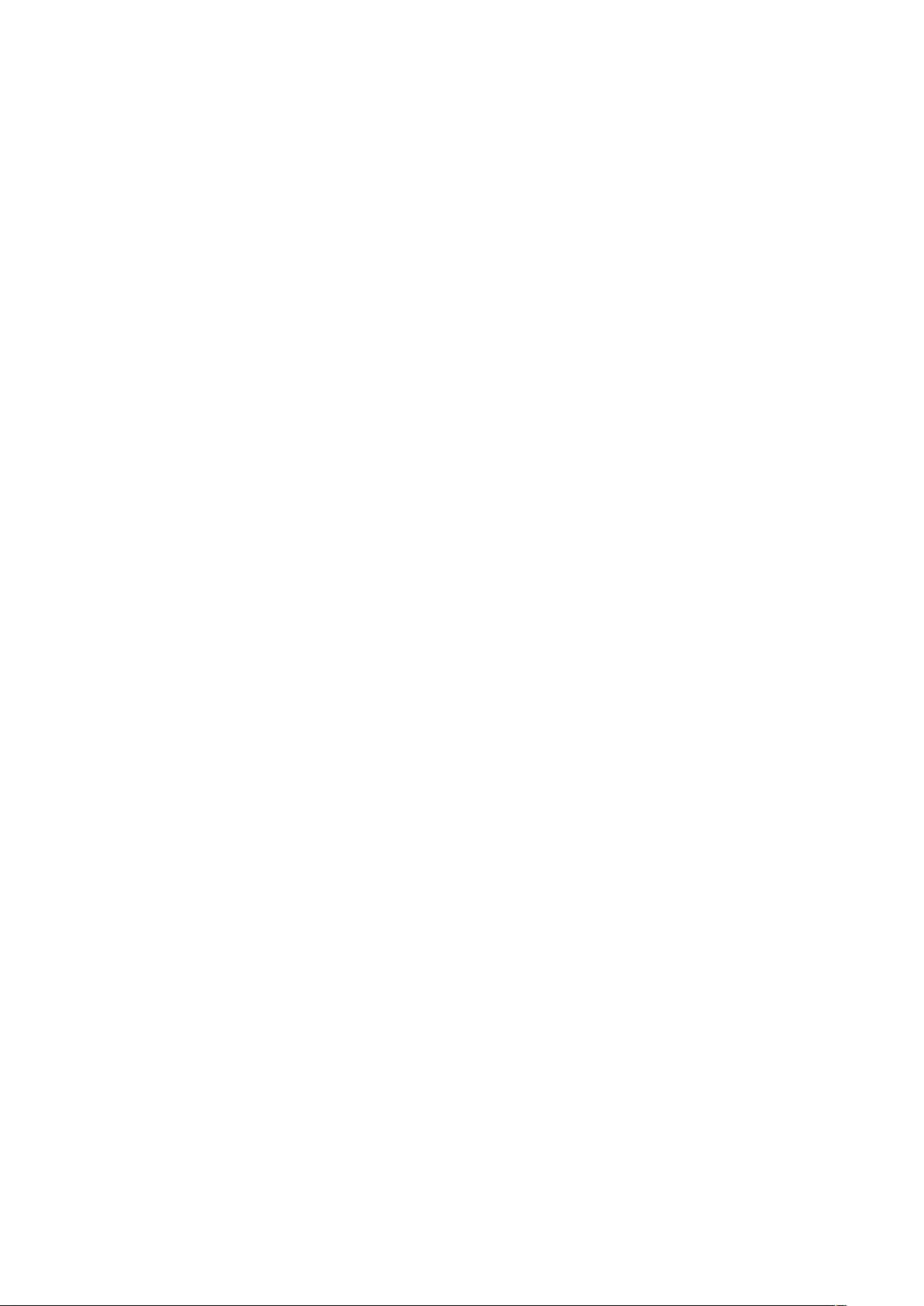
Q, 43
RCA, 32
re-name inputs, 23
reciprocal peak/dip filter, 43
reflections, early, 45
reverberation, 48
role, 14
room modes, 47
S/P-DIF, 33
volume control via Power Link, 25
sampling rate converter, 32
sensitivity, 14,16,28
signal detection, automatic, 24
sound design, 19,29
sound enhance, 18
speaker distance, 14
speaker group, 20
speaker level, 14
speaker preset, 21
speaker role, 14
specifications, technical, 31
SRC, 32
stereo imaging, 7,12
technical specifications, 31
temperature, 27
thermal compression compensation, 30,55
thermal protection, 29
threshold, detection, 24
time-out, 24
treble, 16
USB Audio, 33
volume, 25
volume
control, 16,28
maximum, 27
startup, 16,27
Wireless Power Link, 33
WiSA, 33
WPL, 33
60